Milk is composed of water, fat, protein, lactose, minerals, and vitamins. Currently, dairy producers in Georgia are paid for the amount of skim milk and fat sold. The price of skim milk has decreased significantly after peaking in 2014, but since 2000, but milk fat prices have increased (Figure 1). Today milk fat represents approximately 50% of the value of milk at today’s prices. If component pricing was adopted, producers would also be paid for milk protein and other solids, and the value of skim milk would be reduced even more. Milk containing higher concentrations of fat and protein produces more cheese, butter, or nonfat dried milk per hundred weight (cwt) of milk. With the continued decline in consumption of fluid milk, more milk will be used to produce manufactured products. For this reason, it is important to improve the yield of milk components to maintain the value of milk. Factors that affect milk composition and considerations for feeding to maintain or improve milk composition will be discussed in this bulletin.
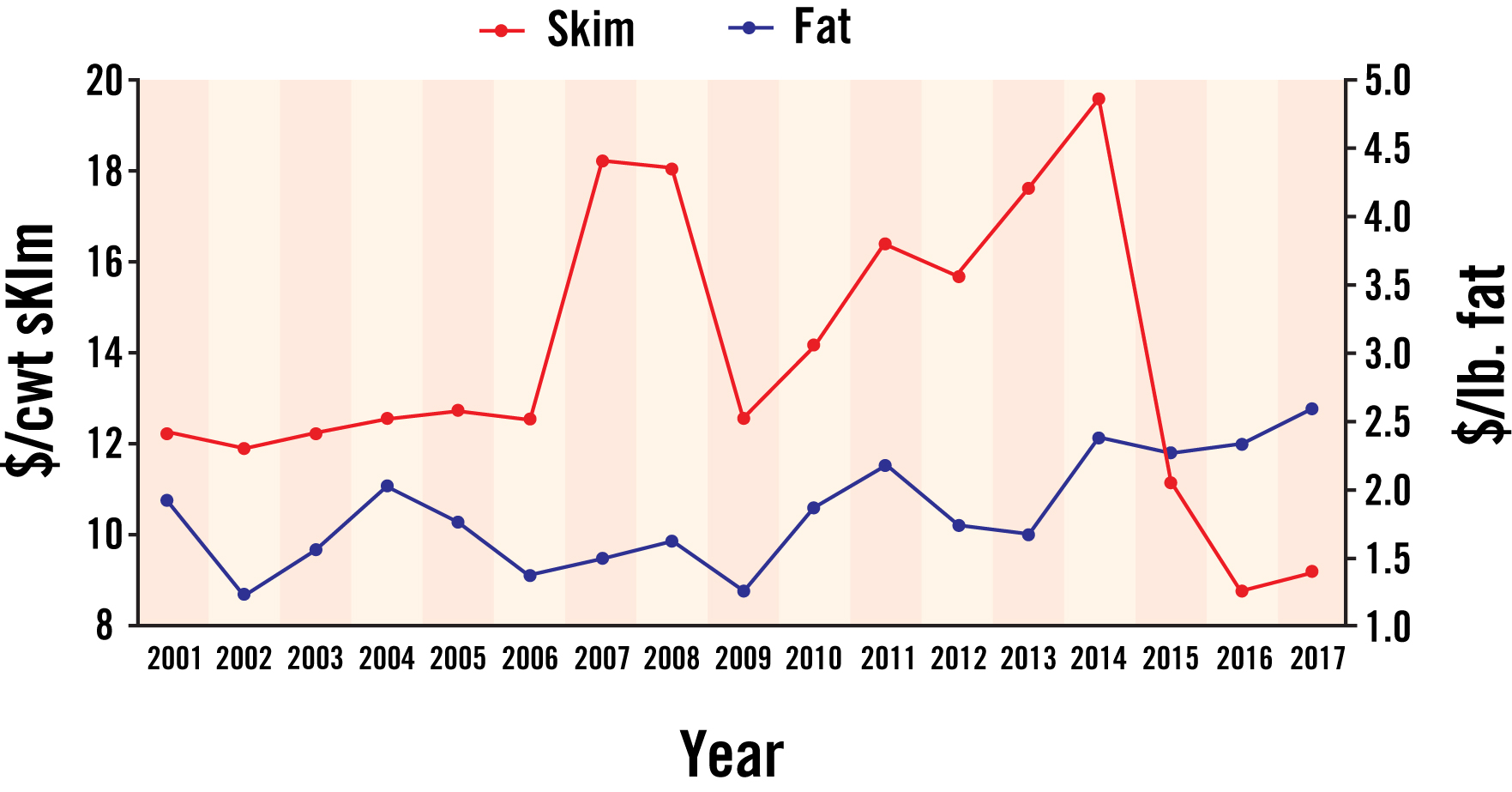
Factors affecting milk composition
Milk fat is the most variable of components whereas concentrations of milk lactose, minerals, and vitamins remain relatively constant under normal circumstances. Milk components differ among the breeds of dairy cows and are highest for Jerseys, intermediate for Ayrshire, brown Swiss, and Guernsey, and lowest for Holsteins. Milk fat and protein are highly heritable traits that are included in sire selection, but it takes several years to significantly change components because of the generation interval of dairy cattle. Herds that have a higher rolling herd average also have a higher milk component yield compared with lower producing herds, which is most likely due to nutrition and management programs. The concentrations of milk components also differ with the stage of lactation, season, and animal health. Milk fat and protein are highest immediately after calving and then decrease during peak lactation and increase near the end of lactation. Seasonally, concentrations of milk fat and protein are highest in the winter and lowest during spring and summer. Cows with elevated somatic cell counts have altered milk composition compared to normal milk as shown in Table 1. Milk fat, total casein, and calcium are lower, whereas whey protein, sodium, and chloride concentrations increase. These changes result in lower yields of dairy products when milk is manufactured, which is why processors insist on high quality milk.
Table 1. The effect of elevated somatic cell count (SCC) on milk composition.
Component | Normal | High SCC1 | % of Normal |
Fat | 3.5 | 3.2 | 91 |
Total protein | 3.61 | 3.56 | 99 |
Total casein | 2.8 | 2.3 | 82 |
Whey protein | 0.8 | 1.3 | 162 |
Lactose | 4.9 | 4.4 | 90 |
Solids-not-fat | 8.9 | 8.8 | 99 |
Sodium | 0.057 | 0.105 | 184 |
Chloride | 0.091 | 0.147 | 161 |
Potassium | 0.73 | 0.157 | 91 |
Calcium | 0.12 | 0.04 | 33 |
1High SCC is considered to be milk with more than 500,000 cells/mL. Adapted from Harmon. 1994. J Dairy Sci. 77:2103-2112
Nutrition and feeding management have the greatest effect on milk composition, especially milk fat. Forty years ago, discussions related to low milk fat percentage would have focused on changes in volatile fatty acid (VFA) production resulting from ruminal fermentation. Milk fat is synthesized from acetate and butyrate, which are produced during the fermentation of carbohydrates. The thinking was that changes in fermentation that increased the proportions of propionate also reduced acetate, which would limit milk fat synthesis. Today we know that the decrease in milk fat is due to incomplete biohydrogenation of unsaturated fatty acids resulting in the formation of trans-10, cis-12 conjugated linoleic acid (trans-10, cis-12 CLA), which inhibits de novo synthesis of milk fat (synthesis of new fat molecules from precursors absorbed from the blood) in the mammary gland (Lock and Van Amburgh, 2012). Normally, microorganisms biohydrogenate, or convert unsaturated fatty acids to saturated fatty acids. This process is illustrated in Figure 2 where linoleic acid, a polyunsaturated fatty acid, is completely biohydrogenated to stearic acid, which is a saturated fatty acid. When the ruminal pH is lower than desirable, an alternate pathway is used for biohydrogenation that results in the formation of trans-10, cis-12 CLA. Incomplete biohydration also happens when the diet contains too much unsaturated fatty acid, exceeding the ruminal microorganisms’ ability to completely biohydrogenate the unsaturated fatty acids (Figure 2, alter BH pathways). The trans-10, cis-12 CLA inhibits de nova synthesis of milk fat by the mammary gland, resulting in depressed milk fat.
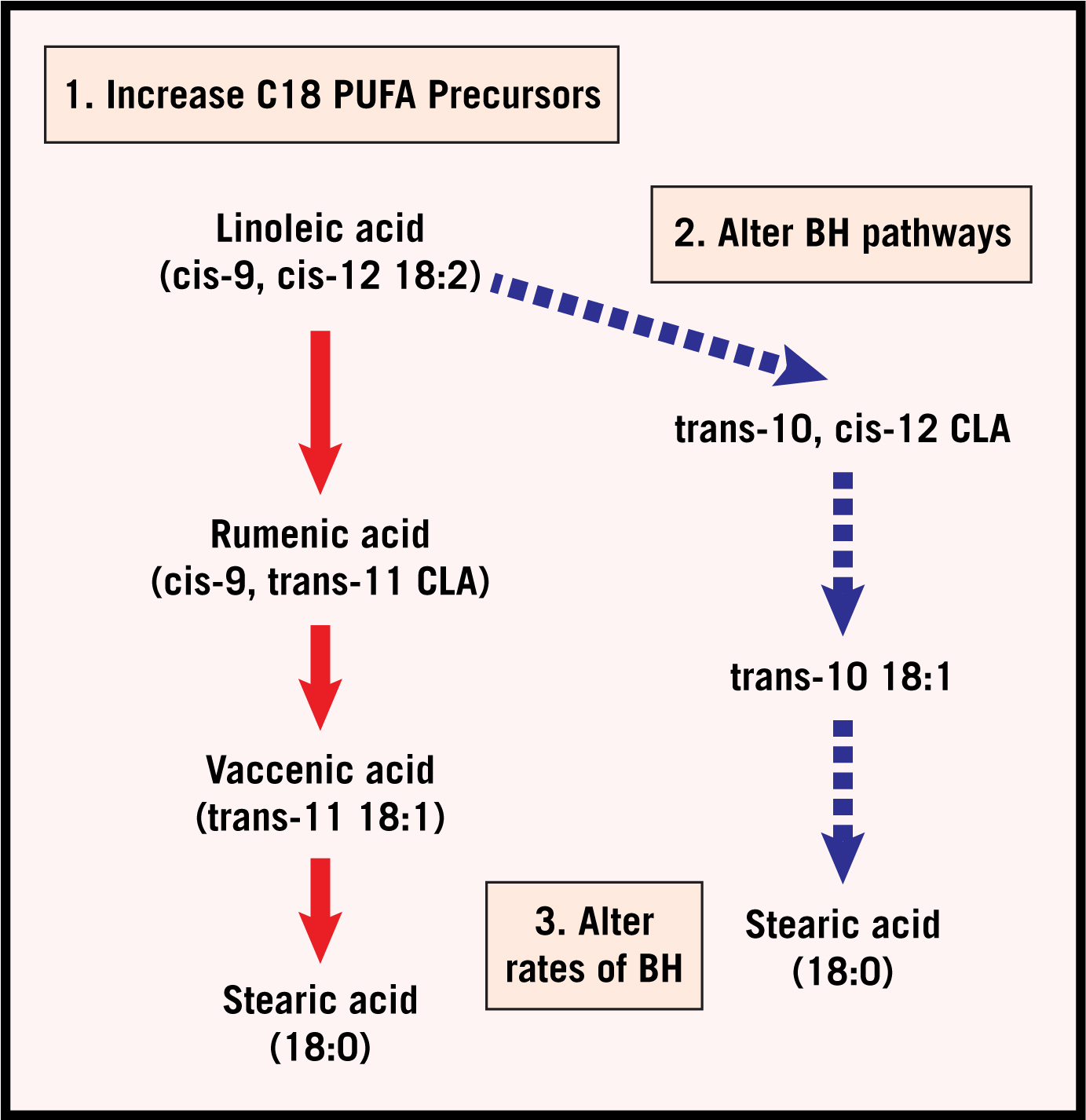
Changes in management that alter eating behavior and resting time have been shown to negatively alter ruminal fermentation patterns, increasing production of trans-10, cis-12 CLA. A recent study identified herds that had high (HDN) or low (LDN) de novo milk fat synthesis (Woolpert et al., 2016). The feeding and management practices of these herds were studied to identify factors that contributed to the differences in milk fat synthesis. The average milk fat percentage and yield for HDN was 4.553% and 2.43 pounds/day, whereas the LDN averaged 4.14% and 1.98 pounds/day. The herds in the study fed diets containing 57.8-58.1% forage. The chemical composition of the diets fed was very similar for both groups and contained 15.1-16.0% crude protein, 22.7-23.7% ADF, 37.4-38.7% NDF, 20.0-23.1% starch, and 8.3-8.9% ash. The HDN diets contained less fat compared with LDN herds, 3.7 and 4.4%, respectively. No differences were associated with feed ingredients or supplements included in the diets. The primary differences observed were associated with management. The HDN herds maintained a lower freestall stocking density (1.05 vs 1.20 cows/stall), tended to provided more bunk space (19.9 vs. 16.7 inches/cow), pushed-up fed fewer times each day (2.7 vs 4.8 times/day), and their cows maintained slightly higher body condition score (3.08 vs. 2.96) compared with LDN. Approximately 80% of the LDN herds fed once each day, whereas only 50% of the HDN fed once daily, which explains the need for more feed push-ups each day and would lead to greater slug feeding on LDN. Improved cow comfort, minimizing crowding, and reducing the change for abnormal feed behavior were positive factors for maintaining higher concentrations of milk components, which is consistent with previous research.
In contrast to milk fat, milk protein synthesis is less variable and primarily dependent on energy supply and a balance of amino acids provided to the mammary gland. Diets that are extensively fermented, without lowering ruminal pH, produce more propionate, which is the primary source of energy and directly related to milk protein synthesis. These diets also optimize microbial protein synthesis. Microbial protein has a very desirable balance of amino acids required for milk protein synthesis. The other source of amino acids used for milk protein synthesis is from the dietary protein that is not degraded by the ruminal microorganisms. The quality of the amino acids from the diet depends on the quality of the original protein in the feedstuff. Undegradable protein sources that contain greater supplies of essential amino acids such as methionine, lysine and histidine are preferable.
Feeding to maintain or improve milk composition
Producers should develop feeding programs that optimize ruminal fermentation and minimize nutrient waste. Most computer programs used to balance diets are based on models that predict the supply of metabolizable energy, metabolizable protein, the amount of polyunsaturated fatty acids, and the supply of minerals and vitamins. In addition to milk yield, these models also predict milk fat and protein composition, as well as ruminal pH. While these models allow more precise diets to be formulated, the feeder has to mix and deliver the diet as formulated for the cow to produce what the model predicts.
Diets should be formulated to avoid decreasing ruminal pH and limit the amount of polyunsaturated fatty acids fed to support normal milk fat and protein synthesis. As illustrated in Figure 3, the amount of forage NDF needed in a diet is affected by several factors. Effective fiber is necessary for maintaining milk fat and
is typically measured by the NDF content of the diet or by the chewing activity of the cow. However, not all NDF stimulates chewing equally. Forage normally is considered to be more physically effective, as measuredby chewing activity compared with high-fiber byproducts that have small particle sizes. The nutritionist must avoid formulating diets with too little forage, feeding too much finely chopped forage, or feeding large amountsof highly fermentable grain. It is also important to avoid feeding excess fat, especially polyunsaturated fat. Some of the byproduct ingredients fed to dairy cattle contain higher concentrations of fat compared with corn or soybean meal. Fat from most plants have higher proportions of polyunsaturated than saturated fatty acids. When forage quality is lower than desired, supplemental fat is often included in the diet to increase the energy density, which may result in depressed milk fat if a ruminally inert supplement is not used. The addition of buffers and other select additives can reduce the potential or extent that ruminal pH is decreased, but these products cannotovercome all potential problems and do not fix the original problem.
There are a number of risk factors in the diet that the nutritionist must account for that can alter milk composition, including low effective NDF, high fermentable starch, forage chopped too fine, high rumen available unsaturated fatty acids, and ionophores (Figure 3). Management practices that alter feeding behavior increase the risk of decreased milk fat. Feeding management practices such as failure to adjust rations for change in dry matter of ingredients, incomplete mixing or overmixing, not pushing up feed, slug feeding, and not feeding for refusals also increase the risk for decreased or depressed milk fat. During heat stress, cows have reduced buffering capacity due to increased respiration, panting, and sweating, which require adjustments in ration formulation, feeding practices, and feeding management to avoid reductions in milk fat.
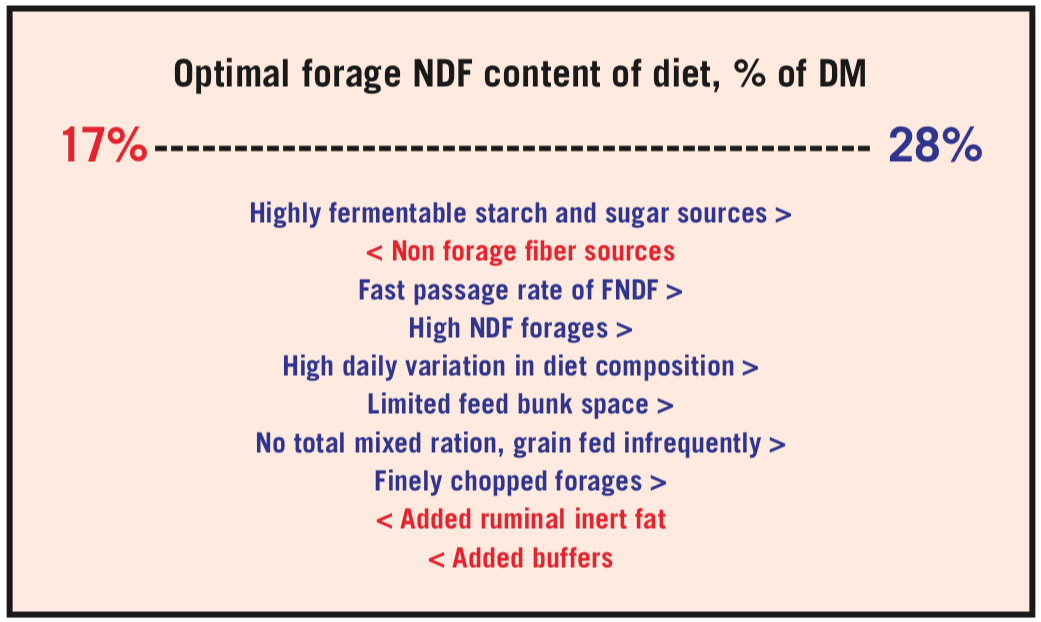
Corn silage is used as the primary forage by most dairy producers. The adoption of kernel processing allows corn to be chopped at a theoretical length of chop between 0.5 and 0.75 inch, which increases the effectiveness of fiber from the corn silage. However, good corn silage contains approximately 50% of its wet weight as grain, which will be rapidly fermented in the rumen when properly kernel processed. Winter annual forages aretypically harvested early in a vegetative stage of maturity, resulting in fiber that is highly digestible but provides less effective fiber than the chemical analysis may suggest, leading to lower ruminal pH and higher passage rate.Feeding supplemental distillers’ or brewers’ grains plus other supplements containing fat may result in higher concentrations of polyunsaturated fatty acids than desired. Replacing some of this forage, distiller’s grains, orbrewer’s grains with soybean hulls or citrus pulp may be beneficial in some cases; however, producers shouldwork with their nutritionist to make changes. Ingredient analysis of feed ingredients should also include fat concentration, rate of digestion, NDF digestibility, and 240-hour undigested NDF to improve the prediction of ruminal pH and effects on milk fat and protein synthesis. Once diets are formulated and presumed to be adequate for supporting normal or improved milk fat synthesis, over processing or underprocessing during mixing or other management factors such as stocking density and feed bunk space may prevent the desiredresponse. This is why it is often difficult to resolve low milk fat issues.
As discussed previously, incomplete biohydrogenation of unsaturated fatty acids leads to the formation of trans-10, cis-12 CLA, which inhibits de novo fatty acid synthesis by the mammary gland. Linoleic fatty acidhas been identified as the primary fatty acid involved in the formation of trans-10, cis-12 CLA; however overfeeding total unsaturated fatty acids can alter ruminal fermentation and must be monitored. The term “RUFAL,” which stands for rumen unsaturated fatty acid load, is the total of oleic, linoleic, and linolenic fatty acids, which are the primary unsaturated fatty acids. The typical concentration of fat, linoleic, and RUFAL composition of commonly used feed ingredients is outlined in Table 2. Compared with animal fat, or tallow, plants have higher concentrations of linoleic acid and RUFAL. The general recommendation for feeding fat is for 2-3% to be provided by base ingredients, 1-2% from supplemental oilseed or tallow, and 1-2% from ruminally inert fat supplements. Ideally diets should contain less than 600 grams/day of RUFAL to avoid milk fat depression.
As outlined in Table 2, many byproduct feeds have higher fat concentrations than corn. Fat from whole oilseed is typically slowly released, allowing it to be completely biohydrogenated, but processing whole oilseed allows the oil to be released quickly. Feeding large amounts of certain byproducts plus oilseed can easily result in dietary RUFAL exceeding 600 grams/day, resulting in milk fat depression. It is rare that one ingredient is the primary problem. Generally, the problem originates in the combination of feeding multiple sources of polyunsaturated fatty acids.
Dietary changes that are used to increase milk protein include optimizing ruminal fermentation and microbial protein synthesis. These changes also improve the efficiency of nitrogen utilization reducing milk urea nitrogenconcentrations. Some opportunities for improving starch digestion from corn based ingredients include
making sure that corn silage is kernel processed completely, storing corn silage for approximately 6 monthsprior to feeding, and replacing cracked or coarsely ground corn with finely ground. If too much corn (starch)is included in the diet, substituting soybean hulls or citrus pulp for corn can help avoid reduced ruminal pH. Protein supplements should complement the primary dietary ingredients to optimize amino acid balance. Diets based on corn silage are considered to be limited by methionine and lysine, whereas diets based on grass are more limiting in histidine. Ruminally protected methionine and lysine supplements are available for feeding when needed to improve milk protein yield, but these should only be used once ruminal fermentation has been optimized as they are more expensive.
Several additives are available that can be used to help stabilize ruminal pH and help prevent milk fat depression. Sodium bicarbonate, sodium sesquinate, magnesium oxide, and potassium carbonate are buffers that improve ruminal pH. These supplements also increase the dietary cation-anion difference that has been reported to improve milk fat percentage and yield. These are most effective in diets based on corn silage or when diets are supplemented with highly fermentable ingredients. Yeast culture has been reported to improve ruminal fermentation and increase microbial fermentation that support higher milk fat and protein yield in some cases (Poppy et al., 2012). Ionophores increase propionate production by reducing methane production (Thomas et al., 2005). These changes in ruminal fermentation improve yield of milk and milk protein and feedefficiency, but can decrease milk fat concentrations in diets containing lower NDF concentrations or excesspolyunsaturated fatty acids.
Summary
There are a number of factors that affect milk composition. Feeding diets that support normal ruminal pH and do not contain excess polyunsaturated fatty acids is very important. Management factors including maintaining good cow comfort, feeding consistency, and adequate feed bunk space also impact milk composition and milk yield. Milk fat and protein yield will be optimized for cows when fed balanced diets that are mixed and fed consistently in facilities that provide optimum comfort.
Table 2. Fat, linoleic acid, and rumen unsaturated fatty acid load (RUFAL) of common forages and ingredients.
Ingredient | Fat | Lineolic | RUFAL | ||
% of DM | % of DM | % of total | % of DM | % of total | |
Forages | |||||
---|---|---|---|---|---|
Corn silage |
3.18 | 1.06 | 33.2 | 1.97 | 52.4 |
Forage sorghum silage | 2.61 | 0.32 | 12.4 | 0.70 | 26.9 |
Oat silage | 3.10 | 0.36 | 11.8 | 1.24 | 40.1 |
Ryegrass silage | 3.08 | 0.31 | 9.9 | 1.01 | 32.9 |
Triticale silage | 2.50 | 0.24 | 9.5 | 1.29 | 51.6 |
Alfalfa hay | 2.43 | 0.25 | 10.2 | 0.77 | 31.7 |
Bermudagrass hay | 1.89 | 0.27 | 14.2 | 0.87 | 46.0 |
Energy supplements | |||||
Corn grain | 3.99 | 2.12 | 53.1 | 3.09 | 77.5 |
Bakery feed | 9.13 | 2.71 | 29.7 | 4.91 | 53.8 |
Brewers grains | 9.53 | 4.09 | 43.0 | 5.36 | 56.2 |
Citrus pulp | 2.87 | 0.60 | 20.9 | 1.11 | 38.8 |
Corn gluten feed | 4.90 | 1.99 | 40.7 | 2.80 | 57.1 |
Distillers grains with solubles | 14.54 | 6.48 | 44.6 | 9.51 | 65.4 |
Hominy | 4.90 | 2.39 | 48.8 | 3.58 | 73.1 |
Soy hulls | 2.96 | 0.69 | 23.2 | 1.15 | 38.9 |
Protein supplements | |||||
Soybean meal | 2.80 | 1.48 | 53.1 | 2.08 | 74.3 |
Canola meal | 3.30 | 0.53 | 16.1 | 2.42 | 73.5 |
Cottonseed meal | 3.12 | 1.53 | 49.2 | 2.10 | 67.5 |
Whole oilseed | |||||
Cottonseed, whole | 18.63 | 10.31 | 55.4 | 13.13 | 70.5 |
Soybeans, whole | 21.85 | 9.39 | 43.0 | 14.69 | 67.2 |
Fat supplements | |||||
Tallow | 100.00 | 8.98 | 9.0 | 46.11 | 46.1 |
Megalac1 | 84.50 | 5.92 | 7.0 | 36.25 | 42.9 |
Energy Booster 1002 | 98.66 | 5.20 | 5.3 | 6.21 | 6.3 |
1Arm & Hammer Nutrition, Princeton, NJ
2Milk Specialties, Eden Prairie, MN
References:
Allen, M. (2007). Formulating Lactating Cow Diets for Carbohydrates. Page 41-50 in Proc. Penn State Dairy Cattle Nutritional Workshop. State College, PA.
Harmon, R. (1994). Physiology of Mastitis and Factors Affecting Somatic Cell Counts. Journal of Dairy Science,77(7), 2103-2112. doi:10.3168/jds.s0022-0302(94)77153-8
Lock, A. L., & Van Amburgh, M. E. (2012). Feeding for Milk Components. WCDS Advances in Dairy Tech. 24:265-277.
Poppy, G., Rabiee, A., Lean, I., Sanchez, W., Dorton, K., & Morley, P. (2012). A meta-analysis of the effects of feeding yeast culture produced by anaerobic fermentation of Saccharomyces cerevisiae on milk production of lactating dairy cows. Journal of Dairy Science,95(10), 6027-6041. doi:10.3168/jds.2012-5577
Thomas, E. E., Green, H. B., McClary, D. G., Wilkinson, J. I. D., McGuffey, R. K., Aguilar, A. A., & Mechor, G. D. (2005). Effect of Rumensin on performance parameters of lactating dairy cows nine-trial registration summary. Page 50-59 in Proc. Florida Ruminant Nutr. Symp. Gainesville, FL.
Woolpert, M., Dann, H., Cotanch, K., Melilli, C., Chase, L., Grant, R., & Barbano, D. (2016). Management, nutrition, and lactation performance are related to bulk tank milk de novo fatty acid concentration on northeastern US dairy farms. Journal of Dairy Science,99(10), 8486-8497. doi:10.3168/jds.2016-10998
Status and Revision History
Published on Jun 07, 2019
Published with Full Review on Apr 04, 2024