INTRODUCTION
In the state of Georgia, where most vegetables are watered using drip or overhead sprinkler irrigation systems, irrigation scheduling is important to ensure that crops are receiving the necessary amount water only when needed in order to avoid crop water stresses and maximize yield (Dukes et al., 2010).
Vegetable crops may experience water stress in two different ways: one, when there is a deficit of water (drought stress), or two, when there is an excess of water (waterlogging or soil water saturation). A water deficit is caused by poor timing of application or insufficient volumes of water applied, limiting soil water availability to plants.Excess soil moisture may be caused by long periods of irrigation or excessive application rates of water, which waste water and induce nutrient leaching. Crop water stresses may also affect crop management. If the soil moisture falls below a critical point where plants are unable to draw water into the roots, wilting will occur. Additionally, any moisture-activated pesticides and nutrients will not be effectively utilized by plants under low moisture conditions.
Currently, there are several different methods of irrigation scheduling (Table 1) that may optimize water application to plants. These methods are ranked according to the level of management required for water application. This publication focuses on water management levels in vegetable crops. It contains basic information of vegetable water use and irrigation management that will assist in irrigation scheduling.
Table 1. The 0-5 (where 5 is best) ranking of irrigation scheduling methods according to irrigation water management.
Rank |
Irrigation scheduling method |
Irrigation water management |
0 |
“Irrigate whenever” method |
Water is applied with no scheduling. |
1 |
"Feel and appearance" method |
Water is applied according to the irrigation manager’s judgment of the appearance of a soil sample and its comparison to soil reference photos. See "Estimating Soil Moisture by Feel and Appearance" (USDA, 1998). |
2 |
Systematic method |
Water is applied based on time or volume, regardless of weather and soil water conditions. |
3 |
Crop water demand method |
Water is applied according to the crop evapotranspiration (ETc). This method consists of calendar-based scheduling according to previous seasons and should account for rainfall events. |
4 |
Soil water status method |
Water is applied based on soil moisture content, typically by supplying a percentage of soil available water in the crop root system. This method should account for rainfall events. |
5 |
Water budgeting method |
Water is applied based on water budgeting, calculating crop evapotranspiration and soil moisture content in the crop root zone. |
IRRIGATION CONCEPTS
Crop evapotranspiration
“Crop evapotranspiration” (ETc) is a term used to describe two processes of water loss from agricultural areas to the atmosphere. The first process is the evaporation, which is the rate of water loss from the soil surface to the atmosphere. The second process is transpiration, which is the rate of water being taken up by roots from the soil, moving through the plant, and evaporating from the leaves (stomata) to the atmosphere. From an irrigation standpoint, the ETc expresses the volume of water lost from the soil and plant to the atmosphere (inches, centimeters, or millimeters) per unit of time (hour, day, week, month, season, or year).
The ETc (Equation 1) is calculated by multiplying a reference evapotranspiration (ETo) by a crop coefficient (Kc).
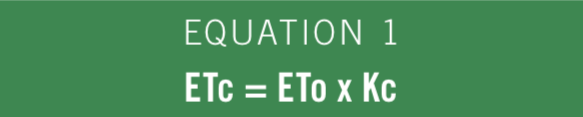
The ETo is the rate of water loss from a soil surface covered with a reference crop via vaporization, according to the Penman-Monteith method (Walter et al., 2000). The Kc represents the plants’ transpiration portion of ETc.The actual daily ETo is available for many locations throughout Georgia through the University of Georgia Weather Network (http://www.georgiaweather.net), which has 85 weather stations installed throughout the state. The Kc values of many crops have been previously estimated (Allen et al., 1998) and will vary according to crop growth stages (Table 2).
Table 2. Crop coefficient values for the initial (Kcini.), mid-season (Kcmid.) and end-season (Kcend) growth stages of the principal vegetable crops grown in Georgia
Crop |
Kcini. |
Kcmid. |
Kcend |
Bell pepper |
0.60 |
1.05 |
0.90 |
Broccoli |
0.70 |
1.05 |
0.95 |
Brussels sprout |
0.70 |
1.05 |
0.95 |
Cabbage |
0.70 |
1.05 |
0.95 |
Cantaloupe |
0.50 |
0.85 |
0.60 |
Carrots |
0.70 |
1.05 |
0.95 |
Cauliflower |
0.70 |
1.05 |
0.95 |
Cucumber |
0.60 |
1.00 |
0.75 |
Eggplant |
0.60 |
1.05 |
0.90 |
Hard (winter) squash |
0.50 |
0.95 |
0.75 |
Onion |
0.70 |
1.05 |
0.80 |
Sweet potato |
0.50 |
1.15 |
0.75 |
Tomato |
0.60 |
1.15 |
0.90 |
Watermelon |
0.40 |
1.00 |
0.75 |
Yellow squash/zucchini |
0.50 |
1.00 |
0.80 |
In the initial stages of crop development, when the crop root system is still shallow and there is a small plant canopy, Kc is generally at its lowest (Figure 1). As the crop leaf area increases, Kc will peak and remain high until the end of the season, when plants start to senesce and there is a decrease in Kc values (Figure 1).
Most vegetable crops have Kc values divided between initial (Kcini.), mid-season (Kcmid.), and end-season (Kcend)values. The largest Kc values are clearly in the mid-season, since the largest crop water requirements occur during this time. Therefore, crop growth stages are key points in irrigation scheduling, ensuring that adequate volumes of water are being applied.
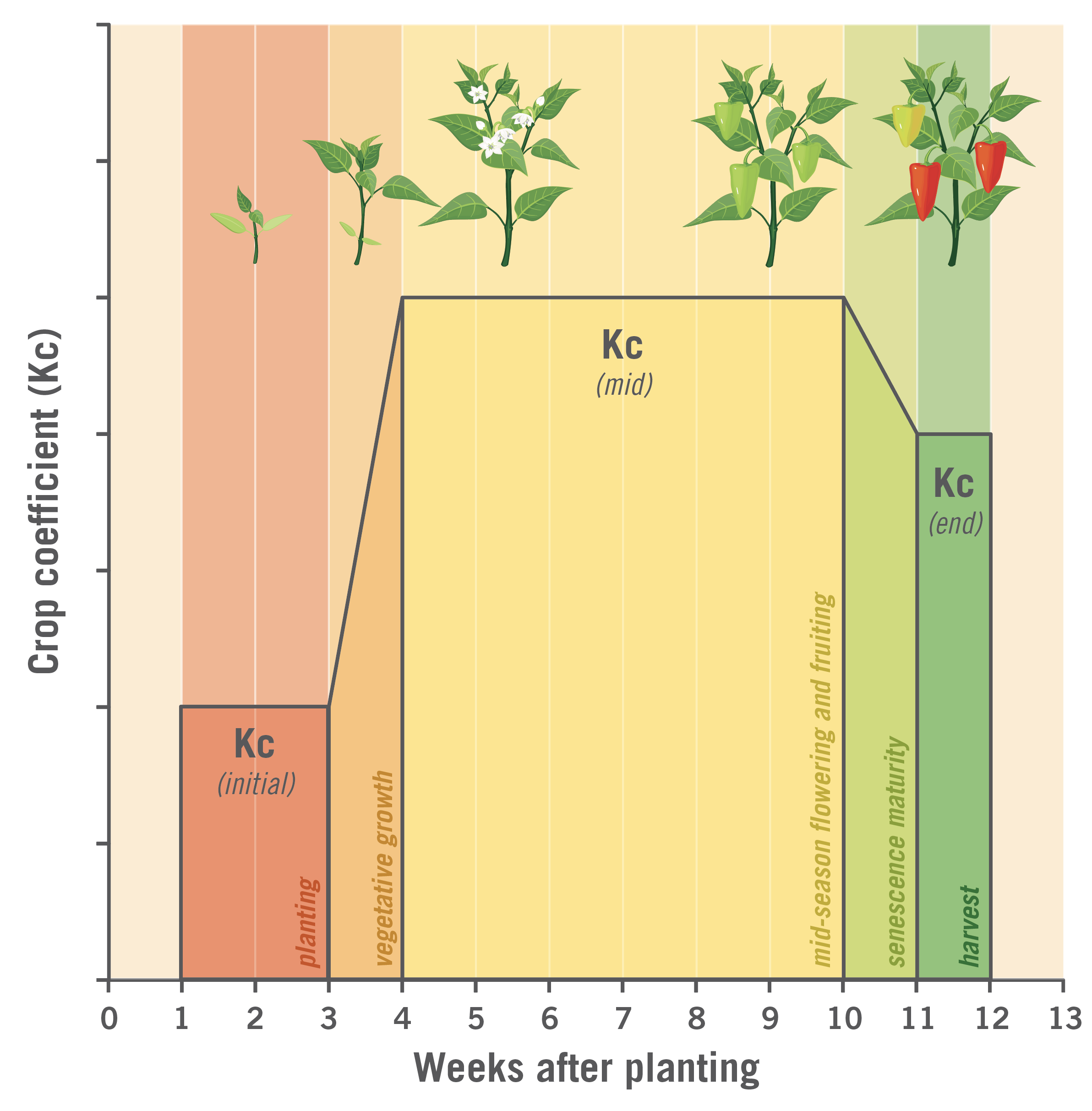
Soil water attributes
It is very important for irrigation managers to understand the characteristics of their soils before establishing an irrigation scheduling program. This optimizes soil moisture content and availability for plant growth and development.
Optimum soil moisture content in the root zone is defined as the ideal water content available for plant uptake throughout the crop season in the established root zone of a soil profile. Different soil types present different capacities to hold water, and soil texture and structure determine the soil water holding capacity. Typically, sandy soils tend to hold less water than clay soils due to the large spaces between the particles and structures present in sandy soils. The water holding capacity of a soil and the ETc demand will determine the frequency of water applications (intervals between irrigation). For example, sandy soils tend to hold less water than clay soil, so sandy soils require more frequent irrigation events than clay soils.
The upper limit of the soil water holding capacity is defined as the field capacity (FC), which represents the fraction of the volume of water retained in the soil after the free water has drained from the soil (Figure 2). During heavy rainfall events, soils may initially be saturated (Figure 2), but after rapid drainage, the soil is no longer saturated (no standing water) and is instead considered at “field capacity.” The lower limit of soil water holding capacity is referred to as the permanent wilting point (PWP). When soils reach the PWP, plants can no longer uptake any water from the soil because the remaining water is bound tightly to the soil (Figure 2). At this point, plants become permanently wilted and may die unless water is supplied immediately. It is important to highlight that if soil moisture contents drop even close to the wilting point, crop yield has already been lost.
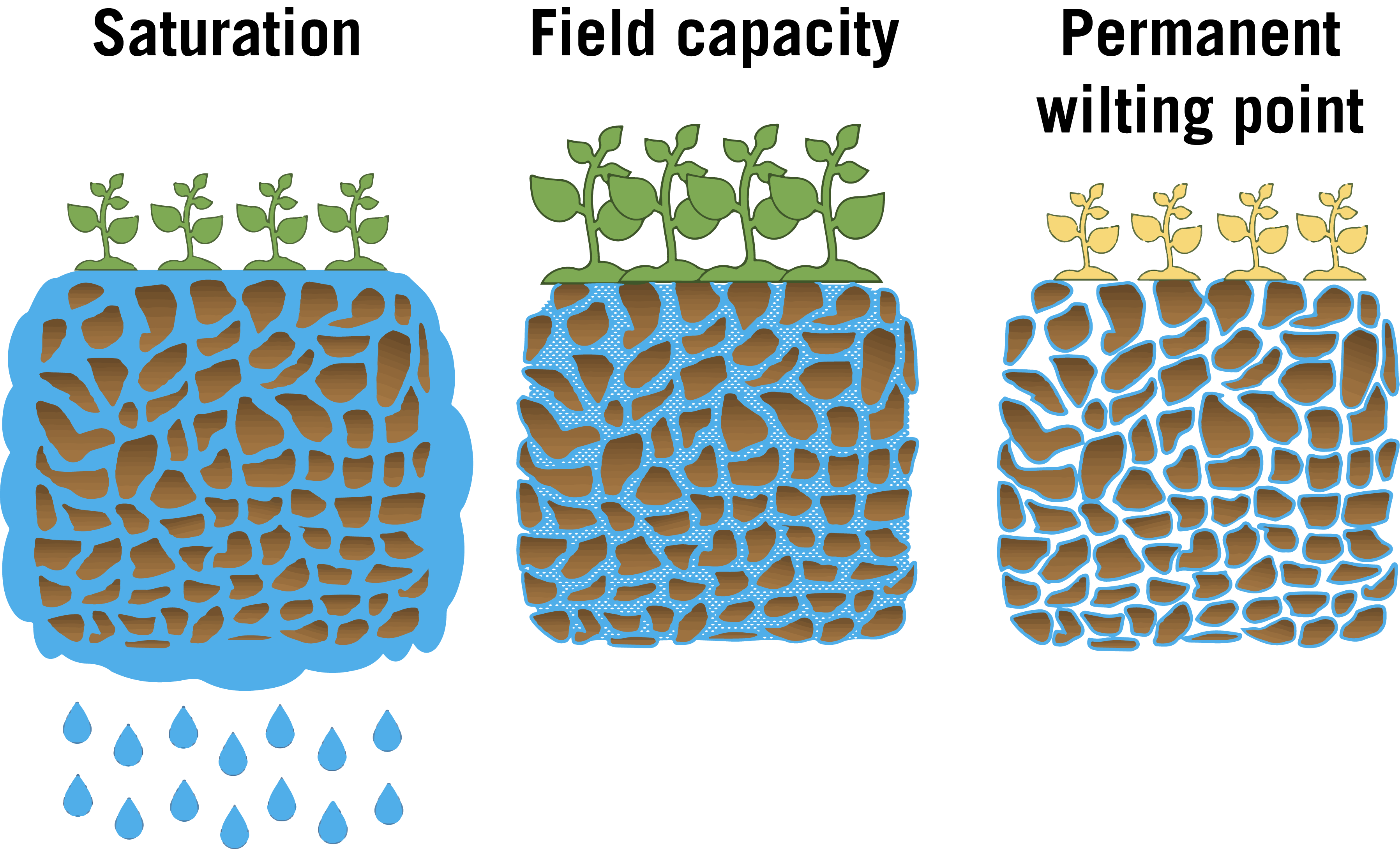
For an irrigation manager, understanding the field capacity (FC) and permanent wilting point (PWP) are important to determining the total available water (AW) in the soil (Equation 2) (Dukes, et al., 2010).
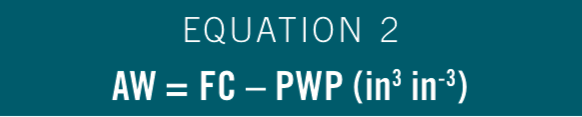
The soil available water multiplied by the depth of the root zone from a selected crop determines the plant available water (PAW) (Equation 3), which is the required volume of water to be replaced if all soil water available to the plant is depleted. Proper irrigation scheduling should never allow a complete depletion of plant available water. Ideally, many vegetables should be irrigated at 70% of plant available water (when 30% of plant available water is used). For the common sandy loam soils found in southern Georgia, allowing soil to dry to less than 70% plant available water can result in yield losses (Figure 3).
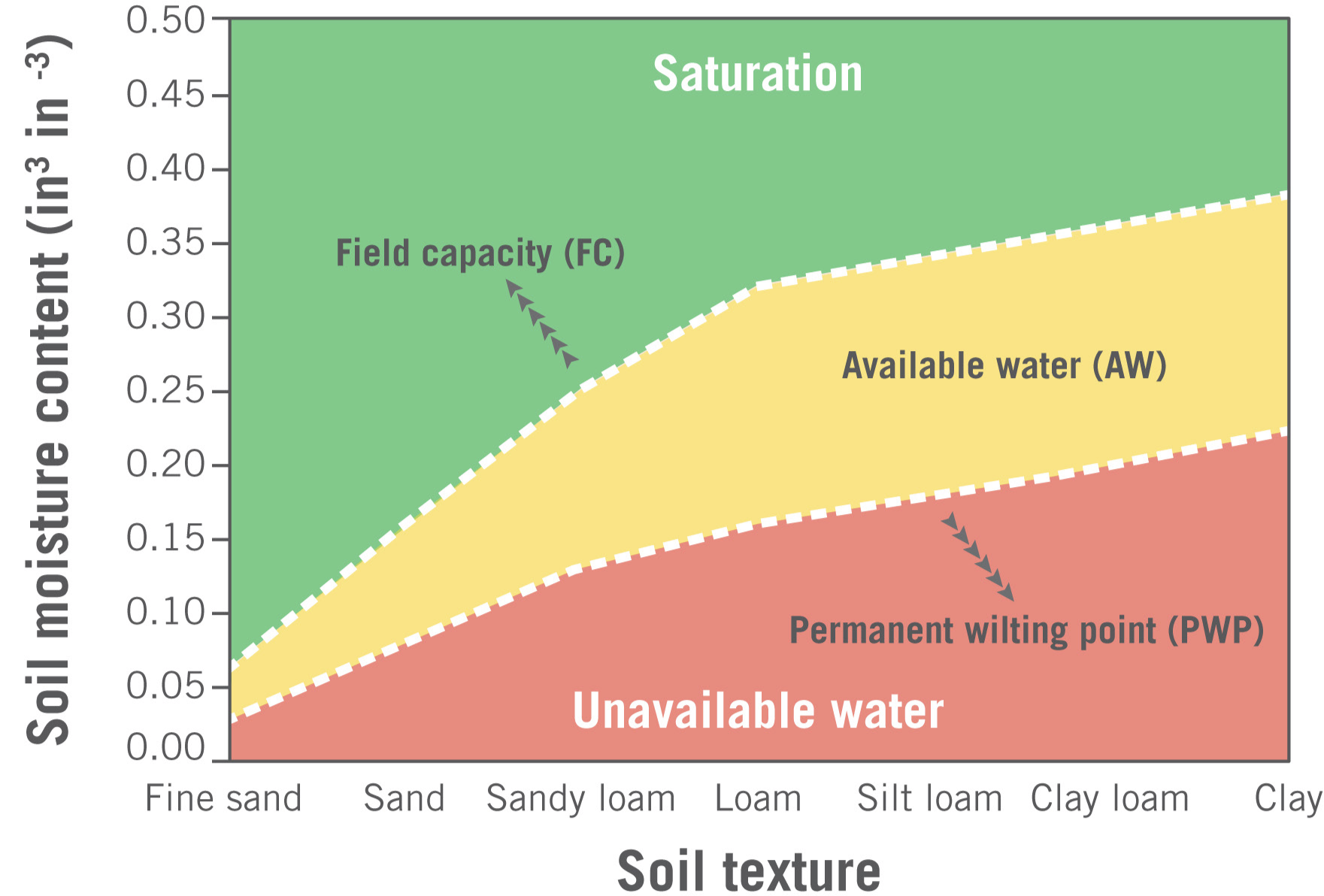

IRRIGATION SCHEDULING
After understanding the crop water demand and soil water attributes, an irrigation manager should select the irrigation scheduling that best fits the crop’s needs and field characteristics. This section describes the fiveirrigation scheduling methods (Table 1) and the water management required for each method.
“Irrigate whenever” method
Irrigating by guesswork is the first and simplest method to determine whether water should or not be applied for a vegetable crop production. This method consists of applying water by guessing how much water should beapplied for a specific crop and when this volume of water should be applied. This method is not recommended,even for those with a great level of expertise in crop and irrigation management.
“Feel and appearance” method
The “feel and appearance” method consists of determining the soil moisture content according to the feel and appearance of the soil. The technique was described by the U.S. Department of Agriculture in 1998, and it is very useful when other irrigation technologies are not available. It can also be used by those with a high level of expertise and experience with a certain crop.
This method requires the irrigation manager to soil sample the crop root zone of an irrigated area using a probe,auger, or shovel. Sampled soil should then be firmly squeezed in the irrigation manager’s hands until it forms a ball or ribbon. From this ribbon, the soil moisture content is determined through comparisons with photos and/or charts (see “Estimating Soil Moisture by Feel and Appearance,” USDA). After the actual soil moisture content is determined, the irrigation manager should estimate the volume of water required to replace the water depleted below soil field capacity.
The “feel and appearance” method also demands experience from the irrigation manager. While this method can be effective for experienced growers, it is not recommended for those growers with little experience producing a new crop on a particular soil type. For example, most vegetable production in Georgia relies on sandy or loamy sand textured soils, with which it is nearly impossible to form a ribbon, making it difficult to estimate the soil moisture content.
Systematic method
The systematic irrigation method for irrigation scheduling is by far the most popular method used for vegetable production in the state of Georgia. This method consists of managing water applications on a time or volume basis, where water is applied every day for the same period of time or in the same amount and time, regardless of crop growth stages or most weather conditions. For example, water is applied for two hours every day with the same flow rate (e.g., from 8-10 a.m. at 1.15 gpm), or there is a daily water application of one-quarter inch.
The systematic irrigation method typically requires a controller standpoint (Figure 4), where the irrigation manager will program the number and length of irrigation events in a daily basis. This irrigation control strategy often avoids crop water deficits, since water will be applied daily. However,the technique does not account for weather conditions (e.g., rainfall) or crop growth stages, which will affect crop water demand. For this reason, waterlogging, nutrient leaching, and runoff are common problems derived from this irrigation scheduling method.
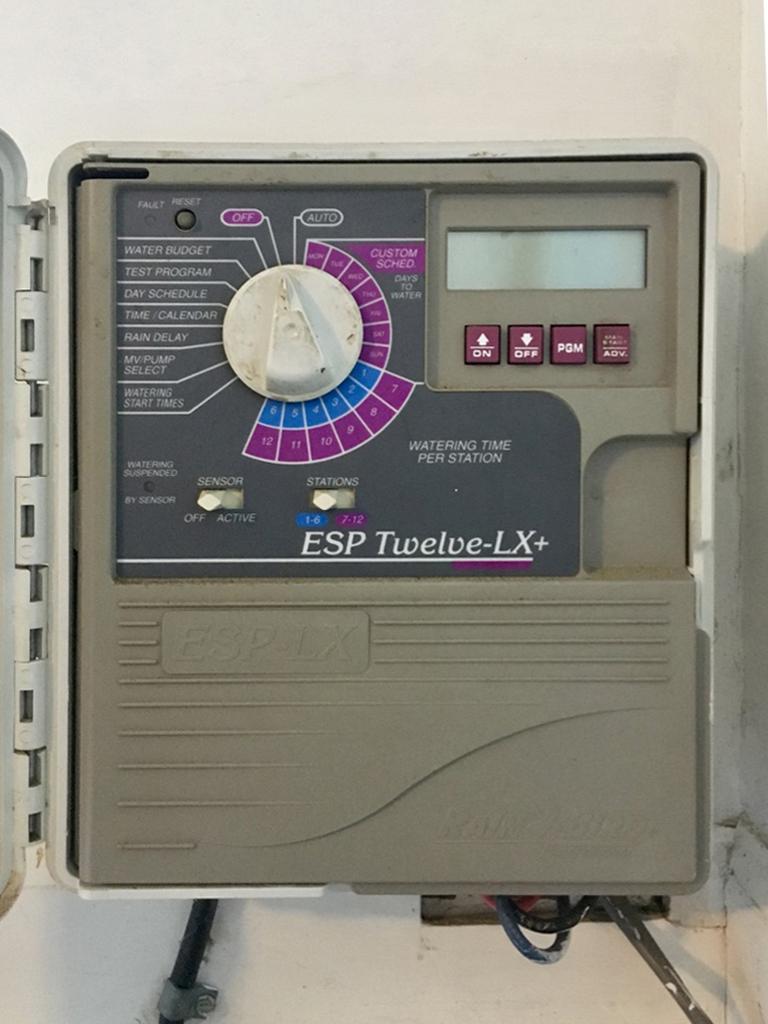
Crop water demand method
Crop water demand is referred to as the “crop evapotranspiration” (ETc). This irrigation scheduling method consists of supplying the daily ETc corresponding to each crop stage of development as discussed previously (Figure 1).
The crop water demand method relies on a calendar-based schedule of irrigation events. This calendar-based schedule can be developed based on previous seasons, where the irrigation manager can calculate the average daily ETc of previous years to determine the average volume of water required for each day. Alternatively, the calendar-based schedule can be developed based on the ETc of the previous day, where the irrigation manager calculates the ETc from the previous day to determine the crop water requirements of the actual day. Currently, there are tools and applications (Smart Irrigation apps) available to control the daily ETc and to help determine the depth of water to be applied.
A common practice of irrigation managers that opt to use the crop water demand method is to determine the average historic ETc for each week of the growing season, and then split that amount of water up over seven days in a week or over two to three large irrigation events during the week. Figure 5 shows the daily ETc for bell peppers in each week of crop development. The daily ETc was averaged from 2000 to 2017 for southwest Georgia. The planting date was August 1 and the average daily ETc for weeks one and two was 0.11 inches on day one, which would require 0.77 inches of water per week. Water could be daily applied at a rate of 0.11inches per day or 0.22 inches every two days. However, it is important to emphasize that transplanted crops (e.g., bell pepper, cabbage, tomato, broccoli, cauliflower, eggplant, and others) will require higher volumes of water and more frequent water applications during the first week of planting. This is important to ensure root establishment. Weeks three and four have the highest ETc during crop development. They require an average of 0.18 inches of water per day. In this case, water application can be scheduled on a daily basis or 0.36 inches every two days for both weeks. Similar approaches should be done for the entire season, but it is recommended that the irrigation manager track plant development to avoid water stress.
In the case of rainfall events, the rain may or may not be added to the water balance for the crop water demand. Research conducted in Tifton, Georgia, has shown that for large raised beds covered in plastic mulch, most rainfall runs off between rows and contributes very little to the actual water needs of the crop. For bare-ground crops, rainfall should be included in the water balance of the crop.
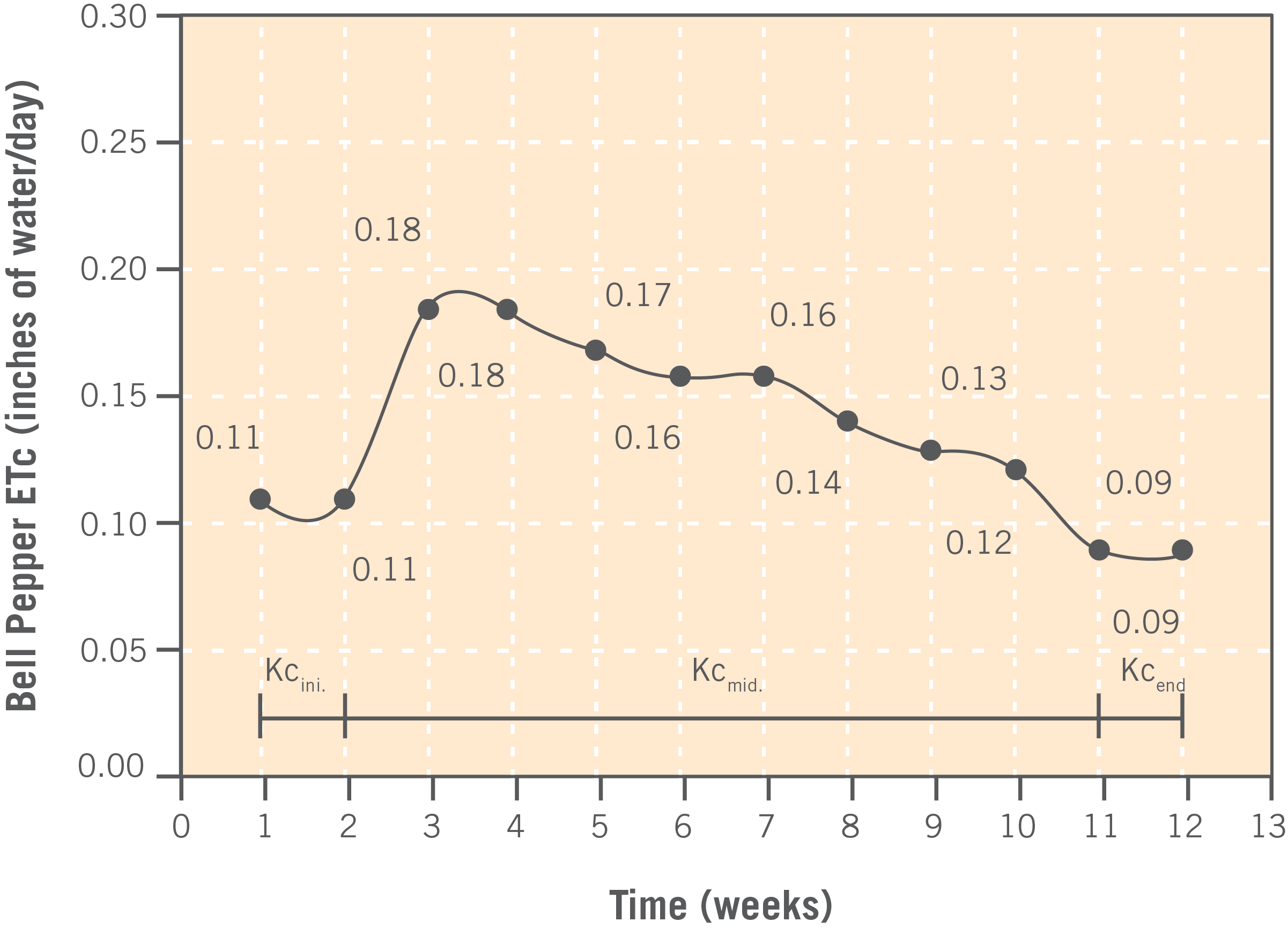
Soil water status method
Irrigation scheduling using the soil water status is also an efficient method. Water is applied to maintain a specific range of plant available water. This method requires sensors to monitor the soil moisture content in the crop root zone, and the volume and timing of water applications are defined by the irrigation manager or by a controller standpoint.
Soil moisture sensors used to determine the soil water status are classified in two categories, sensors measuring the soil water potential and sensors estimating the volumetric soil moisture content. Sensors measuring the soil water potential are also known as tensiometers. Tensiometers measure the amount of tension with which the soil is holding the water. They provide the suction magnitude that plant roots require to uptake water from the soil. The drier the soil, the higher the suction needed. Most vegetable production in Georgia relies on sandy and loamy sand soils, so an irrigation manager should maintain a soil water tension between 6 and 15 cbars. Sensors measuring the volumetric soil moisture content are the capacitance-based sensors, which have increased in popularity in recent years due to their low cost and ease of use. These sensors achieve highly accurate soil waterstatus readings by estimating the volume of water in a specific volume of soil (in3, in-3, or percentage of water content).
Figure 6 is an example of irrigation scheduling using the soil water status method with a sensor measuring the volumetric soil moisture content. The soil moisture of a sandy soil was monitored at a 6-inch soil depth for a period of 50 days. Soil field capacity and permanent wilting point were determined to be 0.16 and 0.04 in3in-3, respectively. Estimated soil available water (AW) is 0.12 in3 in-3, and plant available water at the 6-inch soil depth is 0.72 inches. Irrigation scheduling was set up to maintain plant available water at 70% of AW (the minimum recommended), using the 0.12 in3 in-3 water as the trigger point to irrigate.
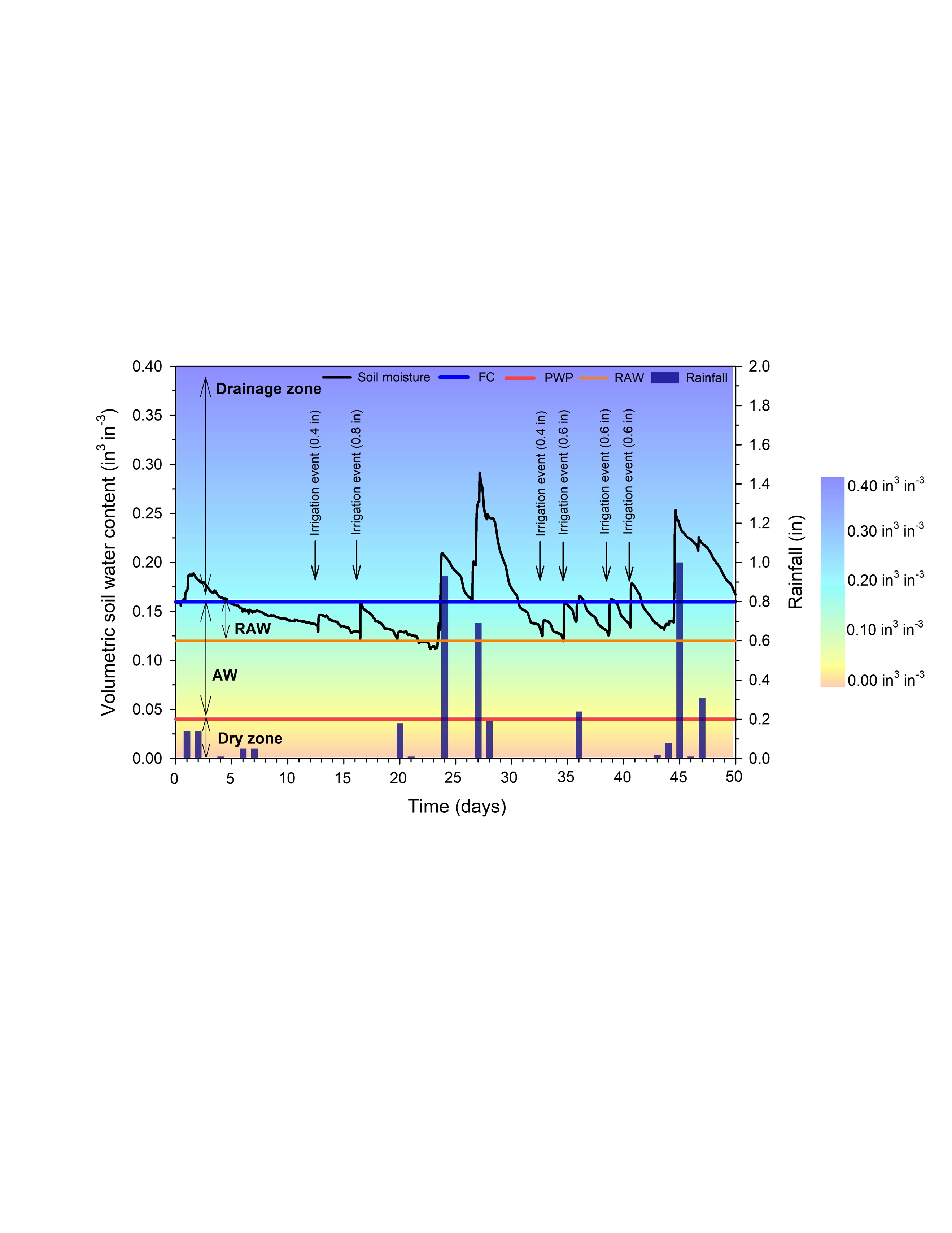
During the 50-day period, overirrigation was avoided, but heavy rainfall events increased the water content in a few points of the season. In the first two days, there were rainfall events that raised the soil moisture content higher than the soil FC (0.16 in3 in-3), and soil moisture content was maintained in the selected range of plant available water (0.12 and 0.16 in3 in-3) for the next 10 days. The first irrigation event occurred at 13 days and 0.4 inches of water was applied to increase the volumetric water content in 0.02 in3 in-3. The second irrigation event (0.8 inches) occurred at day 16 and increased the volumetric soil moisture content from 0.12 to 0.16 in3 in-3. After the second irrigation event, there was a rainfall event of only 0.18 inches (day 20). The rainfall amount was considered minimal, but the volume precipitated held the volumetric soil moisture content in the plant available water range, so there was no water application. Later on, a rainfall accumulation of 1.81 inches between days 24 and 28 increased the volumetric soil moisture content to a status higher than the soil field capacity (0.16 in3in-3) and irrigation events were not necessary for five days. Irrigation events resumed after the excess water was drained (day 32). Soil moisture from day 32 to 41 was controlled by irrigation events and kept within the plant available water range. This period of 10 days when irrigation was the main source of water should be considered good irrigation management.
Irrigation water application in the example above was accomplished manually. There are available automated systems that will irrigate based on water status. These systems can be much more precise and will minimize overirrigation and underirrigation concerns in well-maintained systems.
Water budgeting method
The water budgeting method of irrigation scheduling is the most advanced and is recommended for vegetable production. Irrigation events using this method are adjusted based on the ETc for each stage of crop development (crop water demand method) and corrected using the dynamic water balance required by the soil water status method. In addition, it is recommended that the crop manager develop a rule for splitting irrigation and recording the volumes of water applied.
A rule for splitting irrigation consists of determining a threshold for water application, which may or not vary according to the crop and its growth stages. For example, irrigation events should not apply more than 1 inch of water in a single application or irrigation events should occur every two days. The objective of setup rules for irrigation scheduling is to avoid pushing water from the crop root zone to deeper soil layers. This will avoid soil nutrient leaching as water flows downward thorough the soil profile, reduces environmental impact, and minimizes crop loss.
IRRIGATION EFFICIENCY
Proper irrigation scheduling requires the understanding of crop water demand, soil attributes, and weather conditions. However, delivering the right amounts of water to crops should account for irrigation efficiency.
In an irrigation event, part of the water applied is unavoidably lost during the water delivery to the crop. The volume of water lost will depend on the efficiency of the irrigation system, which will vary among irrigation systems and by system maintenance. Because most of crops in Georgia are irrigated by drip or overhead sprinkler (e.g., center pivot or linear movement) irrigation systems, this section will focus on these irrigation systems, which have an irrigation efficiency of 80% to 90% and 60% to 80%, respectively (Simmone et al., 2004).
Drip irrigation systems, also known as “microirrigation,” operate under low pressure by distributing water via emitters in low flow rates. The water application occurs near the crop root system, reducing water loss by wind drift and evaporation. The reduction of irrigation efficiency in a drip irrigation system is mostly due to inappropriate system design, with incorrect zone sizes, improper emitter placement, pressure losses from friction or elevation changes, and clogged emitters.
Overhead sprinkler irrigation systems lose water during irrigation events mostly due to wind drift and evaporation (Figure 7). To avoid windy conditions and high evaporative demand periods (hot and dry days), water applications should occur in the early morning or late evenings. Water loss is increased with inappropriate nozzles, nozzles applying small droplets, high nozzles, and high angles of water application, which increase the available time for water evaporation and wind drift.
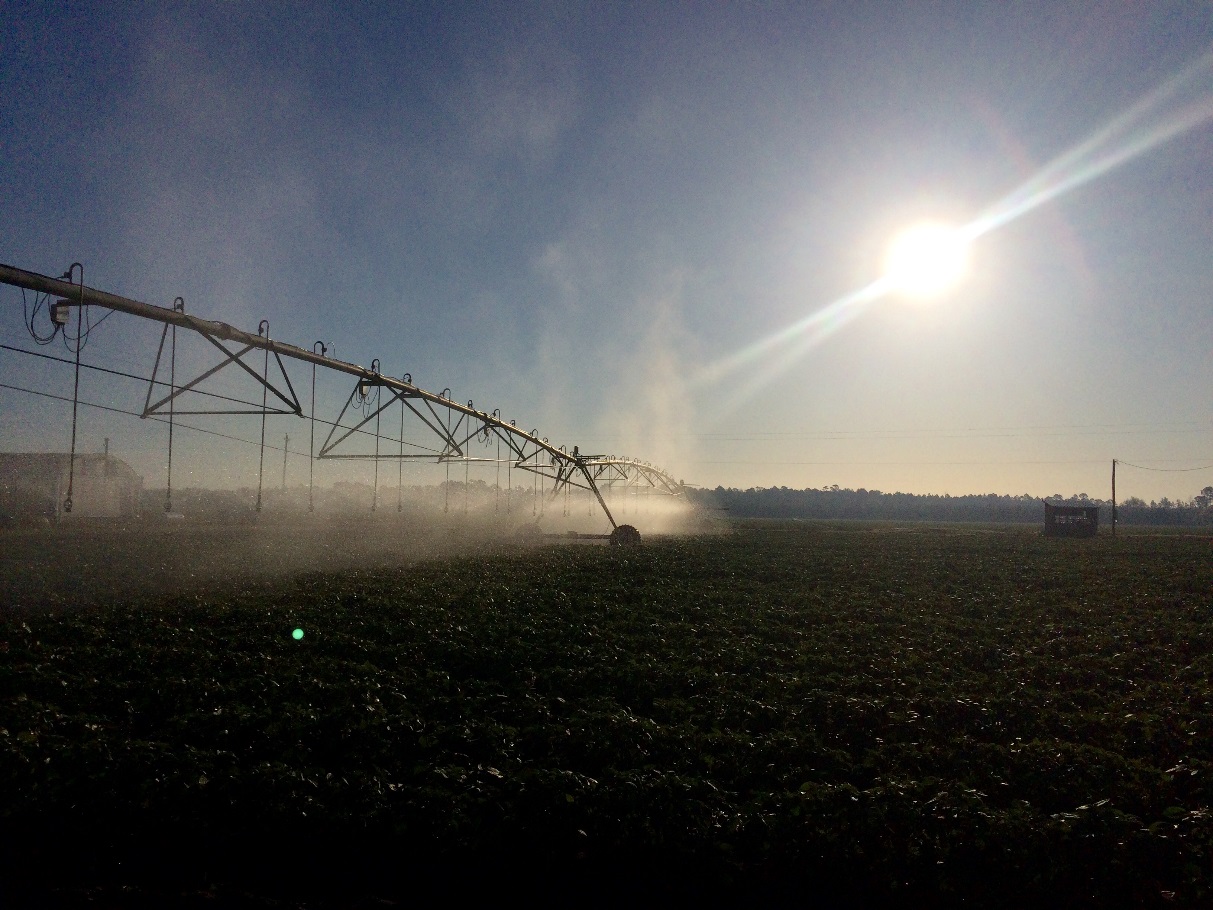
Regardless of the irrigation system, uniform water application is essential to increasing irrigation efficiency. A nonuniform water application can result in overirrigation in some areas of the field, which induce water loss and nutrient deep percolation, and underirrigation (drought stress) in others. It is very difficult to apply water with uniformity whether the system has friction losses, elevation changes, variations in the manufacturing of components, or other factors. Additional information about irrigation uniformity on overhead sprinkler irrigation systems can be found at “Test Procedure for Determining the Uniformity of Water Distribution of Center Pivot and Lateral Move Irrigation Machines with Spray or Sprinkler Nozzles” (USDA, 1989).
SUMMARY
This publication offers a guideline to determine irrigation scheduling for vegetable production in Georgia. Irrigation scheduling varies with water management and growers might require different technologies to properly manage water applications. This information should help the irrigation manager to schedule and operate irrigation systems to optimal capacity, applying water precisely to the crop for maximum effectiveness and high efficiency.
References:
Allen, R. G., Pereira, L. S., Raes, D., Smith, M. (1998). FAO Irrigation and Drainage Paper No. 56. Rome: Food and Agriculture Organization of the United Nations, 56, 97-156.
Walter, I. A., Allen, R. G., Elliott, R., Jensen, M. E., Itenfisu, D., Mecham, B., & Spofford, T. (2000). ASCE’s standardized reference evapotranspiration equation. In Watershed management and operations management 2000, 1-11.
Dukes, M. D., Zotarelli, L., & Morgan, K. T. (2010). Use of Irrigation Technologies for Vegetable Crops in Florida. Horttechnology, 20, 133-142.
Simonne, E. H., Dukes, M. D., & Haman, D. Z. (2004). Principles and Practices of Irrigation Management for Vegetables. Vegetable Production Guide for Florida. University of Florida, Gainesville, FL, 33-39.
U.S. Department of Agriculture. (1989). Test Procedures for Determining the Uniformity of Water Distribution of Center Pivot and Lateral Move Irrigation Machines with Spray or Sprinkler Nozzles. Retrieved fromhttps://www.canr.msu.edu/uploads/235/67987/ASAE_S436.1.pdf
U.S. Department of Agriculture. (1998). Estimating soil moisture by feel and appearance. Retrieved from https:// www.nrcs.usda.gov/Internet/FSE_DOCUMENTS/nrcs144p2_051845.pdf
Status and Revision History
Published on May 31, 2019
Published with Full Review on Mar 03, 2022