- Introduction
- Wheat Growth and Development
- Wheat Variety Selection
- Seed Quality
- Tillage and Wheat Doublecropping Systems
- Wheat Fertilization and Liming
- Planting Wheat
- Weed Management
- Diseases of Wheat
- Insect Management and Control
- Wheat Pest Resistance
- Cropping Systems for Pest Management in Small Grains
- Harvesting, Drying and Storage
- Milling and Baking Quality of Soft Red Winter Wheat
- Production Costs and Marketing Alternatives
- Wheat in Swine Diets
- Small Grains for Non-grain Uses
- Barley
- Oats
- Rye
- Triticale
INTRODUCTION
Small grains — wheat, rye, oats, barley, and triticale — are important agricultural crops in Georgia, South Carolina, Alabama and northern Florida. Winter wheat is a significant cash grain crop in the Southeast, and rye, oats and wheat are planted on hundreds of thousands of acres in each state for winter grazing. Currently, winter barley production is limited, and triticale is a potential cash crop but is not grown extensively in the Southeast.
Small grains are adaptable to multiple-cropping systems, and high grain and forage yields under dry land conditions are attractive features. Wheat is the most important winter grain crop in the southern United States and is typically grown in a doublecrop system with soybean or cotton. Wheat grain acreage in the four southeastern states has been as high as 2+ million acres but currently is about 0.5 to 0.7 million acres, with an estimated gross value of $120 million per year. Furthermore, Georgia is the No. 1 producer of rye in the country.
State average wheat yields have gradually increased over the past 15 years from about 30 to 35 bushels per acre to 45 to 50 bushels per acre. However, a disparity exists between state average yields and yields achieved by top producers, who often reach 80 to 100+ bushels/acre. The potential for greatly increasing production of small grains exists, but better use of management technologies in needed to realize this potential.
The objective of this handbook is to provide rapid and extensive transfer of modern management technologies for small grain production. The intended audience includes Extension agents, industry personnel and dedicated producers. It is multidisciplinary in scope and includes extension and research scientists from agronomy, agricultural economics, agricultural engineering, animal science, entomology and plant pathology from four southeastern states: Georgia, Alabama, South Carolina and Florida.
This is a revision of the Small Grain Handbook originally published by the University of Georgia College of Agriculture in 1989. This handbook revision was developed by the Southeastern Small Grains Team and is jointly published by the University of Georgia and Auburn University Agricultural Experiment Stations and Cooperative Extension Services.
From the Editors:
G. David Buntin
Barry M. Cunfer
WHEAT GROWTH AND DEVELOPMENT
Proper management of wheat for optimum yields requires that certain practices such as nitrogen fertilization and application of pesticides be timed at specific stages of wheat growth. To benefit from these management practices, the grower should understand the growth and development of the plant.
Growing Degree Days
The pattern of development of the wheat plant can be used to guide crop management. Plant development is timed by growing degree days (GDD). Wheat grows and develops when the average daily temperature exceeds 32°F. Wheat development in relation to temperature can be determined by the number of wheat GDD. Wheat GDD are based on Celsius temperatures and are calculated by the formula: average daily (°F) = (max. + min.)/2; then convert degrees F to degrees Celsius (°C) = (5/9) (°F-32). Average daily temperatures less than 32°F are recorded as 32 because this is the lowest threshold for growth and development. Examples of GDD at several average daily temperatures (ADT) are: ADT of 32 = 0 GDD, ADT of 50 = 10 GDD, ADT of 60 = 16 GDD and ADT of 70 = 21 GDD. A GDD calculator can be found here.
Germination
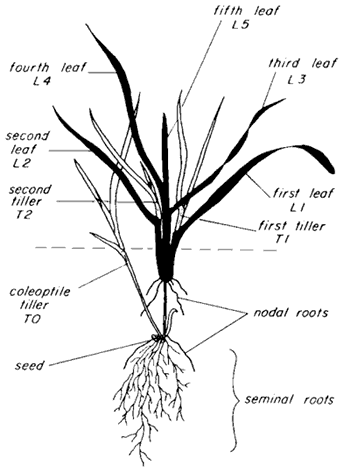
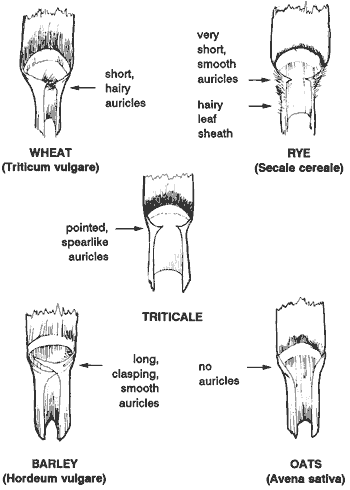
Wheat germinates at temperatures between 39° and 90°F with optimal germination occurring at 68° to 77°F. Germination is indicated by radicle (primary root) protrusion through the seed coat, followed by emergence of the coleoptile (first leaf) which surrounds and protects the emerging stem and primary leaves. Germination normally is complete within four to six days at optimum temperatures. The number of wheat GDD it takes to germinate is 80 and to emerge is 50, for a total of 130 GDD in the Southeast.
Wheat produces a root and crown (nodal) system that develops sequentially according to a pattern typical of grasses. The wheat plant has two types of roots: the seminal and the crown (nodal). Seminal roots form from groups of cells already present in the seed (Figure 1). This system usually comprises three to six main roots and their branches. The first seminal root to appear is called the radicle. It is the first root produced when a seed germinates. The rest of the seminal roots arise from the nodes. Variety and seed size are the main factors that affect the number of seminal roots in the germ.
Crown roots are produced on main stems, primary tillers and secondary tillers (Figure 1). Each main-stem node develops two roots after the leaf first appears. If a tiller is produced at the node, one and sometimes two roots elongate after the first appearance of a leaf at the node. Generally, each leaf takes about 100 GDD to develop.
Crown roots generally begin to grow from tillers after a tiller has at least two leaves. The root axes on tillers elongate in an orderly and predictable way with respect to time. After a tiller has three emerged leaves, the length of roots increases. The depth of rooting increases directly with root number and is also influenced by the soil profile. By the time wheat reaches jointing or early boot stage, new root production ceases, and growth continues in the existing roots. Each tiller has its own roots, which begin to appear when the tiller has two to three leaves.
Seedling Growth
Seedling growth occurs from coleoptile (first leaf) emergence to tiller development. Generally, the wheat plant develops three or more leaves prior to tillering. The rate of individual leaf growth as well as the final shape and size of the leaf are affected by the environment. During vegetative growth, wheat can be distinguished from other small grain crops by its short hairy auricles, which are located at the point where the leaf blade and sheath meet (Figure 2).
Tillering
Tillering is the development of shoots from buds at the base of the main stem (Figure 1). The count of leaves on the main stem is a good way to measure plant development and is linearly related to GDD. Planting to six leaves on the main stem (three tillers) requires about 730 GDD. During initial development, the tiller is dependent upon the main shoot for nutrition, but once the tiller develops approximately three or more leaves, it becomes independent of the parent plant for nutrition and will form its own roots. Varieties show relatively little variation (5 percent to 10 percent) in leaf development rate. Planting date or season-to-season climate variation, appear to create greater change in leaf development rate than variety.
Secondary tillers may also arise from primary tillers. The extent of tillering is dependent upon genetic and environmental factors. Tillering increases with high light intensity, reduced plant populations, and high soil nutrient (primarily nitrogen) availability. High temperatures, high plant populations, soil moisture stress and pests can reduce tillering. Although each tiller has the potential to bear a productive seedhead, generally, about one-half of the tillers do not survive to bear grain. Aborted tillers are affected early in tiller development, long before visual evidence of tiller death is evident.
If a plant that has been stressed during vegetative development is exposed to a favorable environment, rapid tiller growth can improve grain yield. Often low plant populations compensate by increasing numbers of tillers per plant.
Vernalization
The onset of reproduction is controlled by vernalization. Vernalization is the induction of the flowering process by extended exposure of the shoot apex to low temperatures. Vernalization has been shown to occur in seeds as soon as they absorb water and swell. The effectiveness of vernalization declines with increasing plant age. Vernalization is affected by photoperiod, in that exposure of the plant to short days replaces the requirement for low temperatures in some varieties. Also, if wheat is exposed to high temperatures (86°F or 30°C) shortly after low temperatures, vernalization will not occur. After vernalization, the initiation of flowering may be hastened by longer photoperiods, because wheat is a long-day plant requiring night periods to be shorter. Generally, early maturing varieties require fewer chilling hours of vernalization than late maturing ones.
The ability of plants to survive low temperatures depends on whether the plants have been exposed to low temperatures — a hardening process. Later maturing varieties usually survive lower temperatures better than earlier maturing ones. Wheat will go into winter dormancy and grow very slowly when the temperature decreases to 40°F or below. The vernalization requirements for varieties grown in the Southeast range from one day to six weeks.
Jointing
Jointing or stem elongation begins when the first internode of the stem is visible. Generally, wheat stems possesses six internodes, with internodes increasing in length from the base of the plant to the top. Stem height is under genetic control, but the environment affects genetic expression. The end of the jointing stage is indicated by the appearance of the "flag" leaf, which is the last leaf to develop before grain head emergence. From planting to jointing requires about 1,350 GDD.
Boot Stage
Reproductive development is first observed when the head begins to swell within the flag leaf sheath (boot stage). The head is composed of rows of spikelets on the terminal end of the last stem internode (rachis). Each spikelet produces two to five florets, and each floret may produce a single grain. The number of spikelets formed depends upon environmental conditions during early jointing. High temperatures increase the rate of spike development but reduce the number of spikelets per head. Moisture stress reduces spikelet number. High light intensities and optimal nitrogen fertilization increase spikelet numbers. The boot stage ends when the grain head first emerges from the flag leaf sheaf.
Heading Stage
The heading stage is first observed when the head emerges from the flag leaf sheath. Small grains are normally self-pollinated. Pollination begins in the middle region of the head and progresses to the tip and base. High temperatures and drought stress during heading can reduce pollen viability and reduce grain number.
Freezing temperatures may result in head injury and partial or complete sterility during jointing and heading. Few differences among varieties have been found for cold damage during this growth stage. However, short-season (early maturing) wheat varieties are usually more susceptible to injury by freezing temperature because they produce heads earlier in the year than full-season varieties.
Grain Filling
The grain filling stage follows the heading stage. Environmental factors, primarily high temperature and moisture stress, affect kernel survival and the rate and duration of grain development. Starch and protein are the primary storage reserves in the mature kernel. Starch deposition within the grain is under greater environmental influence than protein accumulation. Under high temperature and moisture stress conditions, starch concentration and final grain dry weight are reduced.
Grain Ripening
Small grains are physiologically mature in the hard dough stage. The moisture content may range from 25 percent to 35 percent. The entire plant then loses chlorophyll and assumes a characteristic straw color. At this point, the crop is ready for harvest (13 percent to 16 percent moisture).
Yield is a function of genetics and environment. The yield of a given variety is dependent upon the following yield components:
Tiller no. unit area |
X | kernels head |
X | weight kernel |
= | yield (unit area) |
The factor most directly associated with yield is kernel number per unit area, yet, this factor is dependent upon tiller production, head development, and seed development.
Development Scales
Several small grain development scales have been developed. The two scales most frequently used are the Feekes' and Zadoks' scales (Table 1, Figure 3). The Feekes' scale provides a numerical system for describing wheat growth, but is not very specific during the germination, seedling, jointing, and booting stages. The Zadoks' scale is based on a two digit descriptive system, which allows for more detail in quantifying wheat development. These growth scales allow for comparisons of development among varieties in varying environments and they aid the proper timing of management practices such as nitrogen fertilization and pesticide treatments.
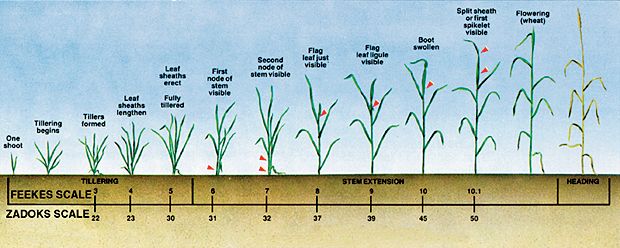
Table 1. Description and comparison of Zadoks' and Feekes' wheat development scales
Zadoks’ Scale
|
Feekes’ Scale
|
General Description | Additional Remarks |
|
|
Germination | |
00
|
|
|
|
01
|
|
|
|
03
|
|
|
|
05
|
|
|
|
07
|
|
|
|
09
|
|
|
|
|
|
Seedling growth |
|
10
|
1
|
First leaf through coleoptile | Second leaf visible (<1 cm) |
11
|
|
|
|
12
|
|
|
|
13
|
|
|
|
14
|
|
|
|
15
|
|
|
50% of laminae unfolded |
16
|
|
6 leaves unfolded | |
17
|
|
|
|
18
|
|
|
|
19
|
|
|
|
|
|
Tillering | |
20
|
|
|
|
21
|
2
|
Main shoot and 1 tiller | |
22
|
|
|
|
23
|
|
|
|
24
|
|
|
|
25
|
|
|
|
26
|
3
|
Main shoot and 6 tillers | |
27
|
|
|
|
28
|
|
|
|
29
|
|
|
|
|
|
Stem elongation | |
30
|
4-5
|
Pseudo stem erection | |
31
|
6
|
1st node detectable | Jointing stage |
32
|
7
|
2nd node detectable | |
33
|
|
|
|
34
|
|
|
Nodes above crown |
35
|
|
|
|
36
|
|
|
|
37
|
8
|
Flag leaf just visible | |
39
|
9
|
Flag leaf ligule/collar just visible | |
|
|
Booting | |
40
|
|
— | |
41
|
|
|
Early boot stage |
43
|
|
|
|
45
|
10
|
Boots swollen | |
47
|
|
|
|
49
|
|
|
In awned forms only |
|
|
Inflorescence emergence | |
50
|
10.1
|
First spikelet of inflorescence | Just visible |
52
|
10.2
|
1/4 of inflorescence emerged | |
54
|
10.3
|
1/2 of inflorescence emerged | |
56
|
10.4
|
3/4 of inflorescence emerged | |
58
|
10.5
|
Emergence of inflorescence completed | |
|
|
Anthesis | |
60
|
10.51
|
|
|
64
|
|
|
|
68
|
|
|
|
|
|
Milk Development | |
70
|
|
— | |
71
|
10.54
|
|
|
73
|
|
|
|
75
|
11.1
|
Medium milk | Notable increase in solids of liquid endosperm when crushing the caryopsis between fingers. |
|
|
Dough development | |
80
|
|
— | |
83
|
|
|
|
85
|
11.2
|
Soft dough | |
87
|
|
|
Fingernail impression not held |
89
|
|
|
Fingernail impression held |
|
|
Ripening | |
90
|
|
— | |
91
|
11.3
|
Caryopsis hard | Difficult to divide by thumbnail |
92
|
11.4
|
Caryopsis hard | Can no longer be dented by thumbnail |
93
|
|
|
Harvest |
94
|
|
|
|
95
|
|
|
|
96
|
|
|
|
97
|
|
|
|
98
|
|
|
|
99
|
|
|
WHEAT VARIETY SELECTION
Well-adapted, high-yielding wheat varieties with resistances to the prevalent diseases and insects are essential for profitable wheat production. Varieties adapted to one area are not necessarily suitable for other areas. Even recommended varieties within a production region vary in yield potential, disease and insect resistance, straw strength, and maturity. Therefore, it is important to carefully evaluate available varieties and plant those that best fit field needs. Errors made in variety selection may result in loss of yield or additional input costs.
Factors to Consider
Yield
Grain yield is normally the primary consideration. Consistent high yields over several years are usually a good indication of well-adapted varieties. However, growers should also consider other factors listed below.
Maturity
Early-maturing varieties ripen about one to two weeks before medium maturing varieties. Early-maturing varieties are most suitable for doublecropping systems. If you are planting a large acreage, use several adapted varieties of differing maturities to increase planting and harvesting efficiency. Late varieties should be planted first, followed by medium, and finally, the early-maturing varieties. If you have to plant later than the recommended date, note that early-maturing varieties usually perform best.
Disease Resistance
Leaf rust, Stagonospora nodorum blotch, and powdery mildew are important wheat diseases. Resistance to these diseases should be considered when selecting varieties. Resistance to leaf rust is most important in the lower Coastal Plain and resistance to powdery mildew is most important under high nitrogen fertility or maximum yield conditions.
Insect Resistance
Hessian fly is the major insect pest of wheat in the Southeast. The most effective and economic means of controlling the Hessian fly is by using resistant varieties. Because Hessian fly populations vary greatly from region to region, varieties reported as resistant in one area of the USA may be susceptible here. See the section on insect management and control for more information.
Straw Strength
Select varieties with good straw strength to prevent harvest losses associated with lodging. Semi-dwarf varieties are short in height and usually have good straw strength, even when high rates of nitrogen fertilizer are used.
Test Weight
Standard test weight for U.S. No. 2 soft red winter wheat is 58 pounds per bushel (lb/bu). Test weights below this standard can result in a price dockage at the elevator. Light wheat with a test weight of 48 to 52 pounds per bushel has low feeding value and lower energy content due to increased fiber content. Environmental conditions, disease and insect damage, and variety strongly influence test weight.
Selecting Varieties for Forage Production
Make sure that varieties selected for forage production have a record of high forage yield. Varieties that produce high grain yield do not always produce high forage yield. Varieties that steadily produce forage throughout the season may be more desirable than varieties with only seasonal growth. If you plan to use wheat for forage and also produce a grain crop, select varieties with high yield potential for both forage and grain production. Use a Hessian fly resistant variety to reduce damage associated with early planting required for fall and early winter forage production.
State Wheat Performance Tests
Each year variety performance tests are conducted to determine the adaptability of available and prospective wheat varieties. Both forage and grain tests are conducted at locations that represent all the major production regions. These results are presented annually in agricultural experiment stations publications and are available at your local Cooperative Extension office by late August each year. These performance data help you judge the merits of wheat varieties.
How to Use the Small Grains Performance Tests Report
- Select the test location that best represents your production area.
- Use multiple year averages, because they are the best predictors of performance.
- Select current varieties you are familiar with and use them to compare other entries.
- Identify those varieties that out-yielded your current varieties.
- If the yield difference between varieties is greater than the LSD value, the varieties are considered statistically different in yield. Yield differences smaller than the LSD value may be due to random variability rather than actual variety differences.
- Check the other columns for test weight, lodging, height, winter survival and heading date. Avoid varieties with low test weight, high lodging or low winter survival. Be cautious about any wheat variety that heads substantially earlier or later, or is significantly taller or shorter than your current variety.
- Refer to supporting tables presenting variety characteristics for general ratings of agronomic characters, disease resistance, and insect resistance.
- Once you have identified one or more varieties that you would like to try, refer to the table titled "Sources of Seed" and contact the variety source for information on availability of seed.
Your soils and management may differ from those of the test location. Therefore you may first wish to plant strips or a small acreage of the better-performing varieties before planting large acreages of them.
Recommended Varieties
Recommended varieties are determined by a critical evaluation of variety performance by research and extension scientists. Recommendations are based on the relative performance of a variety for three or more years, taking into account the importance of diseases, insects, and weather conditions in each of the production regions.
For a list of recommended varieties in your production region, consult the Cooperative Extension fall planting schedule, available at your local Extension office.
SEED QUALITY
Selecting high-yielding varieties and high-quality seed is one of the most important planning decisions in the planting of small grains. Most economic analyses list seed costs as less than 10 percent of total production costs. On the other hand, seed quality and variety selection determine yield potential and many times are the difference between profit and loss.
Seed quality is a collective term to describe the expected performance of a seed, bag of seed or seed lot. It includes standardized measurements of germination percentage, genetic purity, other crop seed, weed seed and inert material. The term seed quality may also include non-standardized measurements of physiological health, vigor and the presence of pathogenic organisms. The standardized measurements are routinely determined by state or private seed laboratories and are required to be listed on each seed tag. The non-standardized measurements are normally used for quality control to select superior lots of seed for sale. However, the non-standard measurements are also available to individuals by special request and need.
Commercial Seed Classes
Farmers can be best assured of high seed quality by purchasing their planting seed from reputable seedsmen. Seed purchased and/or planted by farmers can be placed into one of three classes: certified, non-certified and farmer-saved seed.
- Certified seed, produced by professional seedsmen who are members of crop improvement associations, is distributed by commercial seed dealers. Seed certification is a third party, limited generation, quality control program covering seed fields, conditioning plants, and other critical areas. A major role of the certification program is to deliver new varieties with superior disease resistance, yield, or quality characteristics to commercial growers. The certification staff monitors the seed multiplication process and verifies that the production has met the criteria necessary to protect the genetic identity of new varieties. In addition certification ensures that the genetic purity of older, but highly productive varieties remains stable and the variety remains true to type. While varietal purity is the first consideration in seed certification, other quality standards are important including germination, weed seed, other crop seed, and inert matter content. Certified seed must meet significantly higher standards than is required by state seed laws.
- Non-certified seed also is distributed by commercial seed dealers, but usually do not have third party verification of quality. Seed companies with large breeding programs do not always participate in certification, but many times establish company seed standards which are usually higher than the minimum standards of the state seed law. Non-certified seed may or may not be grown by professional seed producers, but seed quality is known and labeled according to state laws.
- Farmer-saved seed is saved by a farmer to plant on their farm or to sell to a neighbor. They are not required to meet any provisions of the state seed law, provided the seed are sold at the farm, not advertised, or transferred by public carrier. Labeling of farmer to farmer seed is not required. Therefore, little if any recourse is available if the seed fails to produce a stand. Numerous seed surveys conducted by extension and research personnel across the United States report farmer-saved seed consistently produced lower yields. Weed seed contamination, low germination, and unknown or mixed varieties generally lead to the lower yields and lower profits.
State Seed Laws
State departments of agriculture regulate labeling of commercial seed. Seed laws are designed to prevent the misrepresentation of seed and set minimum standards for important seed quality criteria (Table 2). They require all seed offered for sale to be labeled with an analysis tag. Any accredited seed laboratory can determine the seed quality data for the analysis tag. However, the data collected by state departments of agriculture from official samples determine final suitability for sale in the individual states. In addition to the state departments of agriculture, the Federal Seed Branch also regulates seed sales, but it is concerned only with seed traded across state lines.
Table 2. Comparison of analysis requirements as percentage of net weight for classes of seed (Example: wheat, state of Georgia)
Category |
Certified | Non-certified |
Farmer saved |
Pure seed | Minimum 98% | Minimum 90% | No requirements |
Inert matter | Maximum 2% | Maximum 10% | |
Other crop | Maximum 10/lb. | Maximum 5% | |
Weed seed (common) | Maximum 10/lb. | Maximum 2% | |
Germination | Minimum 85% | Minimum 70% |
A typical seed tag provides the following information:
-
Kind and variety
The kind of a crop refers to the species (wheat, oats, barley, rye, etc.) The variety is a subdivision of a kind characterized by growth, yield, fruit or other characteristics that differentiate it from other plants of the same kind. Maturity date, cold tolerance, and disease and pest resistance are variety specific. Contamination of one variety by another may compromise the desirable characteristics of superior varieties. Therefore, genetic purity of planting seed is an extremely important quality factor. Knowledgeable and expert farmers select genetically superior varieties and buy professionally grown seed to ensure varietal purity and trueness to type.
-
Germination percentage
Germination is the ability of a seed to produce a normal seedling under favorable conditions. The laboratory analysis report of percent germination is based on pure seed and not on the total contents of a bag. Actual seed germination potential is greatly influenced by weather conditions during the growing and harvesting seasons. Drought during the growing season may result in immature seed, whereas excessive rainfall may increase disease levels. The storage environment, moisture, temperature and presence of insects also will determine final seed quality. Mechanical damage will cause low seed quality and is caused by improperly adjusted combines, poorly maintained augers, excessive drying temperatures, and high speed impact on hard surfaces in elevators, bins, and trucks.
-
Pure seed
Pure seed percentage gives the percentage of total weight of each kind and variety tested. If more than one kind and/or variety is named, the pure seed percentage of each component must be listed. High quality small grain seeds should always be at least 98 percent pure seed and of one kind and variety.
-
Inert matter
Inert matter includes sand, stones, dirt, sticks and plant parts including pods, chaff and broken seeds. A broken seed is considered inert if it is 50 percent or less of a whole seed. Broken seeds greater than 50 percent of a whole seed are included in pure seed percentage and are considered whole seed for germination purposes. High quality, professionally grown and conditioned small grain seed should have below 2 percent inert matter.
-
Other crop
Other crop may be a contaminant of another kind of small grain or a different variety. In some instances the other crop may be a weed in small grains, but an economically important crop in another planting environment (i.e., ryegrass, cheat, clover or canola). These other crop seed may suggest poor quality controls in seed production and conditioning. Crop plants other than the intended kind may reduce yield and grade and should be avoided.
-
Weed seed
The presence of weed seed is expressed in percentage of total bag weight. It includes seeds, but also bulblets of wild garlic and plant parts of wild radish. High quality seed should contain no weed seed.
-
Noxious weed seed
Noxious weeds are plants that are extremely difficult to control and prolific seed producers. Weeds are declared noxious by state departments of agriculture in a cooperative effort to limit their spread and economic damage. See Table 3 for a listing of the noxious weeds as declared by regulations of the Georgia Department of Agriculture. Other states have similar lists. High quality seed should not contain noxious weed seed.
Table 3. Comparison of noxious weed seeds allowed in various classes of seed
Noxious weeds | Certified | Non-certified |
Farmer saved |
Field Bindweed | Certified seed CANNOT contain any noxious weed seed. | Prohibited | This seed may contain any amount of noxious weed seed. |
Hedge Bindweed | Prohibited | ||
Nutgrass | Prohibited | ||
Cocklebur | Prohibited | ||
Purple Moon Flower (Giant Morning Glory) | Prohibited | ||
Balloon Vine | Prohibited | ||
Tropical Soda Apple | Prohibited | ||
Blessed Thistle | 9 per pound | ||
Wild Onion and/or Wild Garlic | 27 per pound | ||
Sandbur | 27 per pound | ||
Johnsongrass | 100 per pound | ||
Wild Mustard and Turnips | 27 per pound | ||
Blue Weed | 200 per pound | ||
Wild Radish | 27 per pound | ||
Dodders | 100 per pound | ||
Canadian Thistle | 100 per pound | ||
Quackgrass | 100 per pound | ||
Russian Knapweed | 100 per pound | ||
Bermudagrass | 300 per pound | ||
Cheat Or Chess | 300 per pound | ||
Darnel | 200 per pound | ||
Corncockle | 100 per pound | ||
Horsenettle | 200 per pound | ||
Purple Nightshade | 200 per pound | ||
Buckhorn Plantain | 200 per pound | ||
Docks | 100 per pound | ||
Giant Foxtail | 100 per pound | ||
Sheep Sorrel | 200 per pound | ||
Red Rice | 300 per pound | ||
Sorghum Almum | 100 per pound | ||
Sum Total Noxious Weeds (subject to above limitations) | 300 per pound |
Other data required on the seed tag gives information about the specific lot of seed and individual containers. Required labeling information is listed below and in the example in Figure 4a.
- Lot number is a designation placed on each container to serve as a reference of lab reports, producer, year of production, etc. The lot number may be a simple numeric designation as "3" or quite complex as R-6036-JC. The latter number can code for several variables useful in quality control and inventory management.
- Net weight is the quantity of seed in the container exclusive of container weight.
- Origin is the state where the seed was grown. Origin may be an important consideration for crop adaptability when purchasing seed.
- Hard seed does not germinate with standard germination tests, but will germinate upon special treatment such as scarification after ripening, etc. Small grains normally do not have hard seed.
- Dormant seed are viable seeds, other than hard seed, which fail to germinate when provided favorable conditions. Dormant seed may normally germinate over time with exposure to proper conditions.
- Test date is the date that the seed laboratory reported the germination test. State seed regulations require that the test date be within nine months of the date of sale, not including the month of the test. Federal regulations require that the test date be within five months of the date of interstate sale, not including the month of test.
- Name and address of vendor refers to the company responsible for the seed in the bag. In most cases, the vendor is the business that conditioned and bagged the seed for sale or the owner of the seed if another party accomplished conditioning.
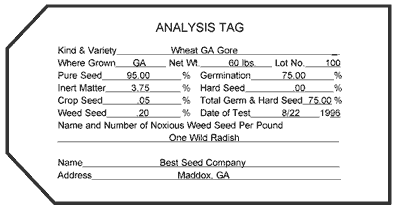
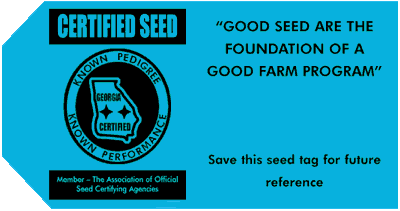
Certified Seed
Every state seed producer has access to a certification agency to assist in the production of high quality seed. These certification agencies administer the production and quality standards established by the membership. The certification label gives the highest level of assurance that the seed in the bag meets the highest quality standards in the seed industry. The certification office also maintains records for each certified seed lot, which includes the following: source of seed planted, application for field inspection and certification, field inspection, bin, conditioning, laboratory report and a sample label from each lot of seed.
The state crop improvement associations were first organized to educate seed growers and consumers about the advantages of high quality seed and provide assurance of seed quality in the bag. During recent seasons and years, with the advent of the Plant Variety Protection Law (PVPA) and expansion of private breeding programs, many private breeding companies have relied on certification for third-party, unbiased quality control. Certified seed is recognized as the best assurance for quality control from the field to the bag. Certification identifies member seedsmen as supporters and providers of high quality planting seed.
Membership in the state crop improvement association is available to qualified seed producers who desire to make certified seed growing a part of their operation. The local county Extension agent can provide appropriate information, addresses and telephone numbers.
There are four classes of certified seed: breeder, foundation, registered and certified. These classes are designed as a four-generation seed increase program to reduce genetic drift and the risk of mechanical contamination. The specific standards for each crop are more restrictive in the foundation class than the registered class and less restrictive in the certified class. In all cases, the standards for foundation and registered seed are established to ensure that the certified class has a high level of genetic purity. Each class of certified seed is described in detail as follows:
- Breeder seed are produced under the direct supervision of the originating plant breeder or institution.
- Foundation seed are grown from breeder seed. Foundation seed are tagged with a white label. The production of foundation seed is highly specialized and usually managed by an organization that has foundation seed production as its primary responsibility. Private companies usually have a specific department for foundation seed production. Foundation seed lots do not normally enter the commercial seed channels but are used by professional seed producers to produce registered and certified seed.
- Registered seed are grown from foundation seed. Registered seed are tagged with a purple label. Recently private and public breeding programs are eliminating the Registered class to provide greater assurance of varietal purity and to limit the unauthorized production and sale of PVP protected varieties.
- Certified seed are grown from foundation or registered seed. Certified seed also are inspected and must meet state seed certification minimum standards. These seed with known pedigree and performance are distributed by commercial seedsmen. Certified seed are tagged with a blue label (Figure 4b). The familiar blue tag found on each bag of certified seed assures the farmer that the seed have passed an unbiased field and laboratory inspection process.
Selecting Good Seed
Yields and profits are greatly dependent on the proper selection of planting seed. Many quality factors must be considered before purchasing seed. An acceptable level for each quality factor must be established.
Perhaps the first and most important decision to make is selection of an adapted variety. The choice of variety sets the upper limit on yield and quality. Trueness to type and varietal purity is just as important as selecting a high yielding variety name. By purchasing professionally grown seed, especially certified seed, the farmer has more assurance of receiving the correct variety with high varietal purity.
Be a seed tag reader. The seed tag reveals the quality of the seed so that the farmer can make a rational decision. It is best to purchase seed with germination above 80 percent, pure seed content above 98 percent, low inert matter and preferably no weed seed. Other crop seed should be less than 0.10 percent.
Remember, cheap seed is usually cheaper for a reason. Seed offered for sale without a seed tag may have a quality problem that could result in poor stands, noxious weeds or a lower yielding variety than the one selected. Saving a few dollars per acre at planting may result in lower or no profit at harvest.
Small grain seed should be treated for diseases and insects. If appropriate chemicals are used, seed treatment will control loose smut and will give partial to complete control of other seed-transmitted small grain diseases. In addition, seed treatments also are important in reducing seedling disease caused by soil fungi. State law requires that all seed commercially treated be tagged with a warning label stating the chemical used and application rate.
Other Considerations
All seed producers and especially farmers who save their own seed or sell seed to other farmers should be aware of the Plant Variety Protection Act (PVPA). The PVPA allows the owner of a variety to determine who may market or sell a variety for planting purposes.
For varieties protected under the 1970 PVPA (normally those varieties developed between 1970 and 1995), a farmer may save the seed from a crop of a protected variety for his own use or sell to another farmer, if he does not normally grow seed for sale and the amount of seed saved is no more than the amount needed to plant his normal acreage.
The 1994 PVPA amended the original PVPA and applies to varieties protected after 1995. This act prohibits the sale of seed for planting purposes unless specifically given permission by the variety owner. To determine which act applies, read the analysis tag on the seed planted or contact the seed certification agency or state department of agriculture in your state.
Determining Stand Problems
Each year, county agents, seed dealers and certification agencies receive complaints from farmers who fail to get adequate stands. But, often times, a thin stand under proper management will produce high yields. As a result each individual situation should be evaluated on its own merits to determine if replanting is desirable and also to establish a reasonable cause for poor seedling establishment.
There are several potential causes of poor stands. Usually, the first assumption is low quality seed. In actual practice though, poor seed accounts for only a small percentage of deficient stands. Most stand problems are related to improper planting depth, soil moisture and/or seed-soil contact. In other cases, chemical injury and improper fertilizer placement have resulted in poor stands from otherwise high quality seed. Seedborne and soilborne diseases, as well as soil insects, can cause reduction in stands. Diagnosing and understanding the reason for stand failure is the first step toward correcting the deficiency, whether it is a seed problem or it is a result of the planting process.
If the probable diagnosis of a poor stand is seed related, it is usually best to immediately contact the seed dealer. In addition, you may wish to notify the county agent, certification agency and state department of agriculture arbitration officials if it is a serious problem that may be difficult to resolve. The process of gathering data should begin at the first indication of a poor stand and should include seed source records, photographs and verified stand counts.
The discussion of poor stands usually leads to questions about seed vigor. Seed vigor is defined by the Association of Official Seed Analysts as "those properties which determine the potential for rapid, uniform emergence and development of normal seedlings under a wide range of field conditions." Several vigor tests are available to assess seed quality, but none are sufficiently standardized for seed control or labeling purposes. Therefore, seed control officials and the American Seed Trade Association do not encourage labeling or advertising with respect to vigor. However, seed vigor information when properly interpreted may prove valuable when making management or seed storage decisions.
TILLAGE AND WHEAT DOUBLECROPPING SYSTEMS
Doublecropping winter wheat with soybeans has become a popular and profitable practice in the Southeast. The long frost-free growing season of the region allows farmers to successfully doublecrop, but higher costs, time and labor constraints, and a desire for less soil erosion have all contributed to increased interest in conservation tillage systems for both crops. New equipment technology for dealing with crop residues, both for drills and deep tillage tools, better herbicides, and the availability of herbicide-resistant soybeans have all enhanced the chances for success.
Soil Compaction
Soil particles (various proportions of sand, silt, and clay, depending on the soil type) are grouped together into aggregates. Aggregates that occur normally are called peds. The larger the aggregates, the more pore space there is for oxygen and water, two important requirements for optimum root growth. When physical forces are exerted on the soil, such as tire traffic and/or tillage, aggregates are broken, or are pressed closer together, reducing the amount of pore space. Less pore space means reduced soil water movement, aeration, and nutrient exchange. The result is a denser, more compact soil, making root growth, rainfall infiltration, and drainage more difficult.
Bulk density is an indicator of how dense or compact the soil is, and is measured as grams of dry soil per cubic centimeter of soil volume. The higher the bulk density, the more compact the soil. For soils with traffic from equipment or livestock, or for soils that have been excessively tilled, the bulk density of the various compacted zones may be so high that crops have difficulty achieving good root growth, and are thus more subject to the detrimental effects of pests or weather extremes such as drought.
Controlled traffic systems reduce the effects of wheel traffic on soil compaction. Tramlines are used so that pesticide and fertilizer application equipment is confined to specific lanes across the field. Electronic tramline systems can be installed on drills so that certain seed drops are blocked so some rows do not get seed to create the tramline areas.
Deep Tillage
Soil compaction can reduce crop yields by restricting root growth. If roots are shallow and do not grow into the subsoil or clay of most soils, they cannot access available soil moisture and nutrients. For most soils, especially those in the Piedmont, there will be a shallow compacted zone about 4 to 6 in. deep that is the result of tillage, primarily with the disk harrow. Because the compacted zone is shallow, chisel plowing to a depth of 8 or 9 in. is usually adequate to break it and allow good crop growth under normal rainfall conditions.
Most doublecropping in the Southeast occurs on the sandy soils of the Coastal Plain. These soils are inherently low in fertility and water holding capacity. They are also susceptible to leaching and often experience significant runoff during the growing season when most rainfall occurs as thunderstorms. The organic matter content of these soils is low, usually less than 1 percent. With excessive tillage, the organic matter content is very difficult to improve and may be as low as 0.5 percent in the lighter textured soils. The low organic fraction contributes to poor soil tilth, which can cause lower rainfall infiltration rates as well as soil crusting. The result is reduced crop productivity, especially under conditions of weather stress, of high or low rainfall.
Most upland sandy soils of the Coastal Plain have a compacted zone or hardpan about 6 to 12 in. below the surface and 2 to 3 in. thick. This is called the E horizon and must be broken so that roots can grow into the subsoil or B horizon for top crop performance. The clay has not only additional moisture but also available nutrients such as nitrogen, potassium, sulfur, manganese and boron.
Deep tillage implements like the chisel plow increase wheat yields in soils typical of the southeastern Coastal Plain. However, in the sandy soils with a highly compacted E horizon, the chiselplow's spring-loaded shanks will ride on top of the hardpan without rupturing it. Consequently, the effective depth of chisel plowing is often only 7 to 10 in., rather that the 13 or 14 in. usually needed.
Subsoilers with shanks 20 in. apart or less can be pulled deeply enough to break through most hardpans, but as with chisel plows the deep tillage is done in a furrow-like fashion. With the combined effects of later trips plus the natural effects of reconsolidation, especially during heavy rainfalls, there is potential for compaction to reoccur. Also, subsoilers and chiselplows often leave ridges or a rough surface, which make seeding depth control for wheat difficult.
New Tools for Deep Tillage
Farmers are interested in reducing tillage trips, leaving crop residues for wildlife and to control erosion, while doing a better job of soil hardpan breakage. A number of new conservation tillage/deep tillage implements leave a large percentage of surface residues while essentially breaking compaction zones in almost a broadcast manner. Examples are the Tye Co. and Bigham Bros. Paratill, Worksaver's Terra-Max, and the French Durou plow. The Paratill uses a slanted shank with subsoiler type points and an adjustable shatter plate behind each shank. The shanks slice through the soil at a 45-degree angle, gently lifting the soil and allowing it to fracture along natural cleavage planes. This action loosens the soil along the bottom of the curved shank without disturbing surface residue. The Terra-Max II plow has one shank per row curved in one direction followed in the same row by another shank curved in the opposite direction. The Durou plow has slanted shanks (20 degrees from the vertical) with a 10-in.-long wing attached to the side of the shank. As with the Paratill, these implements lift the soil and drop it with very little surface disturbance. For these plows, however, there is usually a need for a spike-toothed roller mounted to run just behind the shanks. This is because these implements cause some ridges to form behind the shanks, and smoothing or leveling is needed to help assure uniform depth control.
Wings can be attached to conventional subsoiler shanks to enhance hardpan breakage. The DMI and Yetter companies have optional winged subsoilers commercially available.
Farmers who are considering attaching wings to subsoilers should consider the energy requirements for operating these tools. The wider the wing and closer the shank spacing, the higher the energy necessary for proper operation (Table 4).
Table 4. Tractor size (PTO hp) required to pull one row of various conservation deep tillage implements at 4.5 mph (ground speed) and 15 in. deep in three soil types typical of the southeastern Coastal Plain
Implement* |
Soil type
|
Row spacing for broadcast
tillage (in.) |
||
Clarendon loamy sand
|
Dunbar loamy sand
|
Varina loamy sand
|
||
Yetter (w/o wings) |
26
|
22
|
27
|
17-18
|
Yetter (w/4-in. wings) |
36
|
33
|
42
|
24-26
|
Yetter (w/6-in. wings) |
45
|
40
|
49
|
29-31
|
Terra Max I (single shank per row) |
27
|
24
|
28
|
15
|
Terra Max II (double shanks per row) |
34
|
29
|
37
|
30
|
Paratill |
25-26
|
30
|
22-25
|
|
Durou plow |
25
|
26
|
28
|
20-22
|
* Wings mounted on both sides of subsoiler shanks
Source: A. Khalilian, Clemson University.
Draft and Energy Requirements
Draft and energy requirements of tillage tools are important considerations in selecting tillage systems. The draft needs depend on the soil type and condition, tool shape, travel speed, and depth of operation. Tests were conducted at Clemson's Edisto Research and Education Center at Blackville, S.C., to determine the energy requirements of the latest technology in deep tillage tools for three typical Coastal Plain soils.
Soil compaction measurements before tillage indicated that each test site had a hardpan or E horizon at about the 10- to 13-in. depth. Soil resistance to penetration in the Varina loamy sand site was higher than that for the Dunbar and Clarendon loamy sand sites. This resulted in higher horsepower requirements for the Varina loamy sand.
One-pass Wheat Planting System
Conventional tillage wheat planting systems in the Coastal Plain typically involve a minimum of two or three diskings to bury the previous crop residue, followed by subsoiling or chiseling for breaking the hardpan, and smoothing with a log or other heavy object dragged behind the deep tillage tool). Deep tillage and smoothing the seedbed should be done as one operation. Disking after deep tillage recompacts the soil and essentially negates most of the positive effects of the deep tillage trip.
To complete the conventional doublecrop system, the wheat residue is either burned or disked, then there is usually a one-pass subsoil/planting operation for soybeans. Energy consumption is high for this system, especially when considering the tillage operations, plus cultivation trips for soybeans. There are considerable cost inputs for labor, equipment, plus the investment in management time required. In addition, there is little attention given to the potential for erosion and runoff with these conventional systems.
A reduced tillage one-pass wheat/soybean system has potential to save energy, reduce erosion and runoff, lower production costs, provide food and cover for quail and other wildlife, and expand the planting interval.
Percent crop residue ground cover after planting soybeans averaged 35 percent for the two one-pass systems, 79 percent for the no-till system and only 13 percent for the conventional tillage doublecropping system. The minimum for meeting conservation tillage requirements is 30 percent ground cover. The one-pass systems for wheat provide the deep tillage critical for optimum production of wheat and soybeans in the Coastal Plain soils and they conserve surface residues.
Fall deep tillage improves wheat yield. No-till wheat yields with deep tillage (either in the fall or spring) are not lower than with conventional surface tillage. Yield increased 18 bushels/acre in drilled no-till soybeans when the soil was paratilled, both in the fall and spring.
Table 5. One-pass wheat/soybean test results, Edisto REC, Blackville, SC
Fall deep tillage/planting |
Wheat yield (bu/acre)
|
Soybean yield (bu/acre)
|
|||||
1995
|
1996
|
1997
|
30-in. row
|
12-in. row
|
|||
1995
|
1996
|
1995
|
1996
|
||||
Terra-Max II + CU Drill* |
53
|
59
|
69
|
56
|
36
|
60
|
44
|
Paratill + CU Drill* |
50
|
58
|
68
|
55
|
37
|
59
|
44
|
Disk (2x), chiselplow, grain drill |
47
|
55
|
58
|
51
|
33
|
52
|
39
|
No-till (no deep tillage)+ CU Drill |
31
|
36
|
26
|
39
|
30
|
44
|
34
|
* One-pass planting
Source: A. Khalilian, Clemson University
Table 6. Wheat and soybean yields for reduced tillage tests at the Pee Dee REC, Florence, SC
Fall deep tillage (Paratill)
|
Surface tillage (Paratill)
|
Spring deep tillage
|
Wheat yield (bu/acre)
|
Soybean yield (bu/acre)
|
|
30-in. row width
|
7.5-in. row width
|
||||
yes
|
disked
|
yes
|
48
|
37
|
63
|
yes
|
disked
|
no
|
45
|
36
|
53
|
yes
|
no-till
|
yes
|
51
|
43
|
85
|
yes
|
no-till
|
no
|
50
|
39
|
67
|
no
|
disked
|
yes
|
41
|
39
|
59
|
no
|
disked
|
no
|
35
|
36
|
49
|
no
|
no-till
|
yes
|
42
|
41
|
70
|
no
|
no-till
|
no
|
34
|
36
|
52
|
Source: J. Frederick et. al., Clemson University
Conclusions
The new winged plows that break soil hardpans in a broadcast fashion, e.g., Paratill and Terra-Max, will be used especially in the Coastal Plain, when considering conservation tillage and the desired residual effects of deep tillage under controlled traffic systems. Deep tillage implements such as the subsoiler and chisel plow will continue to be used, but as farmers switch to reduced tillage to reduce erosion and costs for equipment, labor and energy, there will be shifts toward the newer drill and deep tillage technology. The new herbicide resistance technology for soybeans (e.g., Roundup Ready) will help enhance the chances for success with reduced tillage and drilled systems for soybeans. Controlled traffic systems using tramlines also will reduce soil compaction.
WHEAT FERTILIZATION AND LIMING
Fertilization and liming are critical management practices for wheat production. A properly managed fertility program, including recommended fertilization and liming practices, can improve yield and quality more than any other single management practice. Such a program includes soil testing, knowledge of crop nutrient requirements and removal, timely application of nutrients, and record-keeping.
Soil pH and Liming
Maintaining proper soil pH is critical to ensure availability and uptake of fertilizer and soil nutrients essential for good wheat production. Economic wheat yields are optimized at soil pH between 6.0 and 6.5. Not only are the essential plant nutrients, including micronutrients, available in this range, but soil elements toxic to plants such as aluminum are kept unavailable.
Liming is the best management practice required to maintain soil pH in the proper range. Lime recommendations should be based on soil testing. It is not uncommon on sandy soils and with modern crop rotations, to need lime applications as often as every year. Dolomitic limestone, which is commonly used, will also provide both essential secondary nutrients, calcium and magnesium.
Nitrogen (N)
Nitrogen rates and timing of application are key management factors for making good wheat yields. Nitrogen is also the most expensive fertilizer nutrient and variable input cost for wheat. Therefore, nitrogen rates should be based on soil potential, cultivar, realistic yield goal, previous crop and residual N. For expected wheat yields of 40 to 70 bushels/acre, use a total N rate of 80 to 100 lb/acre. Adjust this rate based on the preceding crop. If following peanuts or soybeans, decrease the N rate by 20 to 40 lb/acre. If following grain sorghum or cotton, increase by 20 to 40 lb N/acre. Timing of N fertilization should be based on the pattern of uptake by the crop. Demand for N is relatively low in the fall but increases rapidly in the spring just prior to stem elongation. Therefore, apply 20 to 40 lb N/acre at planting, and the remaining N prior to stem elongation. Use the lower rate at planting on heavier-textured soils and the higher rate on sandy soils. Also, excessive N rates applied in the fall could result in a number of problems including surplus vegetative growth, winter kill, disease incidence and possibly nitrate contamination of the groundwater.
When the yield goal exceeds 70 bushels/acre, use a total N rate of 120 lb/acre. Adjust this rate for the preceding crop as above. Also, on sandy soils, use two top-dress N applications, one at early tillering and another at early jointing. This can improve yields when N leaching conditions occur. Although yields may not always be improved, this practice can also reduce the amount of N released into the environment and offers the chance to adjust N rates downward if climatic or economic conditions do not warrant the added expense of the last N application. Adjust N rates up or down as you experiment with you particular farm. Avoid excessive N rates in the spring, as it leads to lodging and a reduction in milling properties and flour quality.
Phosphorus and Potassium (P and K)
Phosphorus and potassium fertilizer applications should be based on soil testing. Because 65 percent of the total P uptake and 90 percent of the total K uptake occurs before the boot stage, these nutrients should normally be applied before planting. Split applications (half at planting and half at topdress) of K is recommended on deep sandy soils (greater than 12 in. to subsoil clay). Use of starter fertilizer containing P, especially in reduced tillage systems, is being investigated but not recommended at this time.
Secondary Nutrients
Calcium and magnesium (Mg) are normally supplied when maintaining proper soil pH with dolomitic limestone. If soil pH is adequate but calcium or magnesium are not, applications of fertilizers containing calcium such as calcium sulfate or magnesium such as magnesium sulfate will be recommended.
Sulfur (S) is an essential nutrient that used to be contained in many other fertilizers and in atmospheric deposition or fallout from smokestacks. Today's fertilizers are largely free of sulfur and sulfur is often scrubbed or removed from smokestack emissions. Therefore, wheat growers now need to make a conscious effort to include at least 10 pounds of sulfur per acre in their fertilizer applications. This can be accomplished either with preplant fertilizer or by including sulfur with topdress N. Because sulfur is mobile in sandy soils, including S with topdress N is the preferable applications timing. In addition, the N:S ratio in plant tissue should be maintained between 10 and 15 to 1 (N:S) and can be easily checked by using plant analysis.
Micronutrients
Micronutrient levels in Georgia's soils are usually adequate for wheat production unless soils have been over-limed. The two micronutrients most likely to be deficient, and the ones routinely tested for with soil testing, are zinc (Zn) and manganese (Mn). Adequate baseline levels of soil test Zn and Mn should be maintained. If low levels of either of these essential micronutrients are detected, low doses in soil-applied fertilizers should be added to build up the soil test levels. Plant tissue analysis should be used in conjunction with these applications, and foliar Zn or Mn should be used if deficiencies are detected by plant analysis. Attempting to correct low soil test levels with soil-applied fertilizers in one application can be expensive and possibly ineffective and therefore are not recommended. Manganese deficiency occurs most frequently in poorly drained soils of the Flatwoods region. Availability of Mn declines significantly as pH increases above 6.2 to 6.5 in these soils. Deficiencies of other essential micronutrients such as molybdenum, copper, boron, iron, and chloride in wheat are rare.
Doublecropping
Other crops, such as soybeans, are often doublecropped following wheat. Fertilizer recommendations are available to try to supply P and K fertilizer for both crops before planting wheat. These recommendations are often based on crop removal of nutrients by both crops. Because fertilizer nutrients such as K and soil pH levels can drop rapidly on sandy Coastal Plain soils, taking a soil sample after wheat is harvested but before the next crop is planted is encouraged if possible.
Nutrient Removal
Nutrient uptake and removal varies with yield (Table 7). Most fertilizer recommendations account only for nutrients removed in the grain. When straw is also removed, additions of P, K and S should be increased for the following crop. In addition, burning wheat straw, which is not recommended due to loss of organic matter that is potentially valuable to sandy soils, returns P and K but not N to the soil.
Table 7. Nutrient uptake and nutrient removal by wheat at different yield levels. Removal based on grain only
Nutrient
|
40 bu/acre yield
|
70 bu/acre yield
|
100 bu/acre yield
|
|||
Uptake
|
Removal
|
Uptake
|
Removal
|
Uptake
|
Removal
|
|
N
|
75 lb/A
|
46 lb/A
|
130 lb/A
|
80 lb/A
|
188 lb/A
|
115 lb/A
|
P2O5
|
27 lb/A
|
22 lb/A
|
47 lb/A
|
38 lb/A
|
68 lb/A
|
55 lb/A
|
K2O
|
81 lb/A
|
14 lb/A
|
142 lb/A
|
24 lb/A
|
203 lb/A
|
34 lb/A
|
Mg
|
12 lb/A
|
NA
|
21 lb/A
|
NA
|
30 lb/A
|
NA
|
S
|
10 lb/A
|
NA
|
18 lb/A
|
NA
|
25 lb/A
|
NA
|
Poultry Litter
Managed properly, poultry litter (manure mixed with bedding material) can be a valuable source of plant nutrients for wheat production. It is most like a complete fertilizer, containing significant amounts of primary, secondary, and micronutrients except for boron. It can be a relatively inexpensive source of P and K, but trying to meet all of the N requirement with poultry litter is not recommended.
On average, broiler litter contains approximately 3 percent N, 3 percent P2O5 and 2 percent K2O (fertilizer value of 3-3-2). Based on this average, one ton of poultry litter contains 60 lb of N, 40 lb of P2O5 and 40 lb of K2O. Based on current fertilizer prices for N, P and K, poultry litter is valued at approximately $25/ton. The nutrient content of litter varies significantly however, depending on moisture content, type of bird, feed ration, and especially storage and handling methods. Therefore, it is highly recommended that litter be analyzed for nutrients by a reputable laboratory before determining application rates and value.
Application rates of poultry litter for fertilizer are usually based on the nitrogen requirement for the crop grown. Poultry litter is also best used as a preplant incorporated, complete fertilizer to supply P, K, secondary and micronutrients to the crop on a timely basis. For wheat, an application of 2 ton/acre of poultry litter (preplant incorporated) will supply an adequate amount of fall N and should meet the P and K requirements of even a soil testing low in P and K. The availability of P and K in poultry litter is considered comparable to commercial fertilizer. For N, it is estimated that only 25 percent of the N in poultry litter is readily available. The remainder is in a slower released organic form. Therefore, excessive N in the fall should not be a problem. Release of adequate amounts of N from litter in the spring will depend on a number of factors, especially weather conditions. At the recommended 2 ton/acre rate of poultry litter, topdress applications of approximately 50 lb N/acre are likely needed. The crop should be monitored in the spring and topdress N applications should be adjusted accordingly.
PLANTING WHEAT
Planting Date
The optimum planting date for wheat depends upon where it is grown and how it is to be used. Table 8 shows the suggested planting periods for both grain and forage production in the four major production regions of Georgia, Florida, and Alabama.
Wheat planted for grazing should be planted earlier than wheat planted for grain. Wheat planted late does not accumulate forage before winter and therefore does not provide good fall grazing.
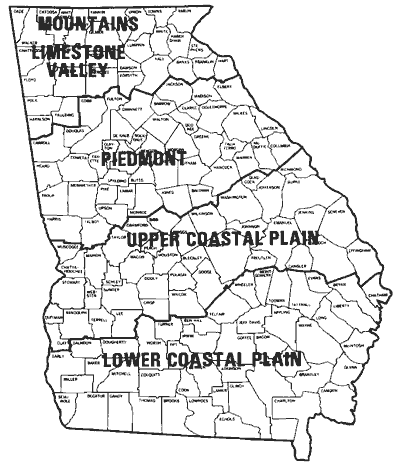
To obtain high yields and good grazing, plant within the recommended planting dates for your area. Acceptable grain yields have been made when planted later, but yield potential is usually reduced when compared to plantings made during the recommended period. Planting during the recommended period provides adequate time for tillering and root development and reduces the potential for excessive winter damage. Although wheat emerges sooner and the shoot (above-ground portion of plant) develops faster in warm soil (75° to 80°F), the root system develops much faster and more extensively if the soil is cool (55° to 60°F).
Wheat planted earlier than the recommended period will be subjected to more disease, Hessian fly damage, and aphids and subsequently to more winter injury. Many wheat varieties require a period of cold temperature (vernalization) before plants form a grain head. Varieties with short vernalization requirements are particularly sensitive to early planting because their vernalization requirements can be met too quickly. These varieties should be planted in the latter part of the recommended planting window.
Planting later than the recommended date can be even more damaging to yield potential. Studies in Georgia have shown planting one week beyond the recommended planting period can reduce yield 20 percent below normal, and planting three weeks late can reduce yield 50 percent below normal. Late-planted wheat will have fewer tillers, be susceptible to winter injury due to a lack of crown development, and develop grain during the warmer, more humid conditions of late May and June. If planted late, the vernalization requirements of some varieties may not be satisfied and the plants will not develop grain heads until late in the season. If planting is delayed more than two weeks beyond the last recommended planting date, use only early maturing varieties with short vernalization requirements.
Table 8. Optimum planting periods
Area |
Grain |
Grazing |
Georgia | ||
|
Oct. 15 to Nov. 1 | Sept. 1 to Oct. 15 |
|
Oct. 25 to Nov. 15 | Sept. 15 to Oct. 15 |
|
Nov. 7 to Dec. 1 | Oct. 1 to Nov. 1 |
|
Nov. 15 to Dec. 1 | Oct. 15 to Nov.15 |
North Florida | Nov. 15 to Dec 15 | Oct. 15 to Nov. 15 |
Alabama | ||
|
Oct. 15 to Nov. 1 | Sept. 15 to Nov. 1 |
|
Oct. 15 to Nov. 15 | Sept. 15 to Nov. 1 |
|
Nov. 1 to Dec. 1 | Oct. 1 to Nov. 15 |
Seedbed Preparation
Wheat performs best when planted on well-drained, fertile soils that have been prepared as a smooth, firm, and weed-free seedbed. Studies show that tillage can greatly affect wheat yields (see the chapter on tillage). Research in the Southeast has consistently shown economical yield responses to deep tillage, especially on Coastal Plain and Piedmont soils, which tend to compact. Deeper tillage allows for easier root penetration, burial of diseased debris, and improved water infiltration. In wet years, low soil-oxygen conditions are enhanced by compacted, dense soils. This condition reduces yield due to detrimental effects on root production a nutrient uptake.
Chiseling, turn plowing, subsoiling or paraplowing results in better yields than disking alone. Pulling a leveling device behind some form of deep tillage such as a chisel plow has been very effective on most soils in the Southeast. Shallow seedbed preparation (accomplished by no more than one disking) is less acceptable on most soils in the region. No-till planting for winter grain production is rarely used in the Southeast.
Planting Method
A grain drill is the best tool for planting wheat. Planting with a drill reduces seed cost, insures better germination, produces more uniform stands, reduces winter injury, reduces competition from weeds, and almost always increases yields. A 10 percent to 15 percent yield increase for drilled versus broadcasted wheat is common.
Although broadcasting and disking can save time, they require more seed per acre and result in many seeds being placed too shallow or too deep for germination and emergence. If you have no choice but to broadcast, increase the seeding rate by 20 percent, set the covering disk to cut about 4 in. deep, and drive at a ground speed of 5 to 7 mph.
Seed Treatments
Fungicide and insecticide seed treatments are an inexpensive but effective method of protecting seed and seedlings from seed and soilborne diseases and early season insects such as aphids and Hessian fly.
Drill-box seed treatments are limited to several types of fungicides. Best performance is achieved when the seed is fully covered at label rates. See the disease and insect sections for specific recommendations.
Seeding Rate
The optimum seeding rate generally varies between 1½ to 2 bushels/acre for grain and 2 to 2½ bushels/acre for grazing. Wheat seed size varies greatly from year to year, among varieties, and between seed lots of a variety. Wheat seed may range from 9,000 to over 20,000 seed/lb. These differences exist because the environment in which it is grown primarily determines seed weight or kernel plumpness. An example is provided in Table 9. Notice the differences between varieties. All of the varieties were grown under the same environment.
Table 9. Seeds per pound and test weight of seven varieties of wheat grown in 1995
Variety
|
Seed/Pound
|
Test wt.
|
Hickory
|
14,232
|
59.0
|
Savannah
|
11,180
|
58.0
|
Coker 9803
|
12,770
|
57.5
|
GA-Dozier
|
14,641
|
55.3
|
GA-Gore
|
12,628
|
57.1
|
GA-Stuckey
|
15,539
|
52.3
|
Morey
|
11,990
|
56.8
|
Average
|
13,283
|
56.6
|
Seeding based on seeds per acre is much more accurate than seeding based on weight per acre. Generally, a seeding rate of 35 seeds per square foot is desirable. Tables 10 and 11 provide examples of planting rates based on seeds per row foot for different row widths and varying seeds per pound. For this reason, it is important to plant on a seed per row foot basis.
Table 10. Seeds per row foot needed to achieve certain seeds per square foot at different row widths
Row width (in.)
|
Seeds/sq. ft.
|
|||
30
|
35
|
40
|
45
|
|
6
|
15
|
18
|
20
|
23
|
7
|
18
|
20
|
23
|
26
|
7.5
|
19
|
22
|
25
|
28
|
8
|
20
|
23
|
27
|
30
|
10
|
25
|
29
|
33
|
38
|
Table 11. Pounds of seed as determined by row width, seeding rate and seeds per pound
Seeds/row ft.
|
6 in. row width
|
8 in. row width
|
10 in. row width
|
|||
12,000
|
15,000
|
12,000
|
15,000
|
12,000
|
15,000
|
|
18
|
130.7
|
104.5
|
98.0
|
78.4
|
78.4
|
62.7
|
22
|
159.7
|
127.8
|
119.8
|
95.8
|
95.8
|
76.7
|
26
|
188.8
|
151.0
|
141.6
|
113.3
|
113.3
|
90.6
|
30
|
217.8
|
174.2
|
163.3
|
130.7
|
130.7
|
104.5
|
If you are fertilizing for a yield of 60+ bushels/acre or you are planting either before or after the recommended period, plant the maximum recommended rate in order to maximize yields. |
Seeding Depth
Under optimum planting conditions, you can plant wheat 1 to 1½ in. deep and expect to produce a good stand. If conditions are dry, plant the seed 1½ to 2 in. deep. The deeper planting depth will require ¼ to ½ in. of rain to germinate the seed and will ensure that enough water is available to the young seedlings to keep them growing once they germinate and emerge.
If wheat is planted deeper than 2 to 2½ in., a large portion of the seedlings may fail to emerge, or they may be abnormal once they do emerge (Table 12). This is because many seed lack the food reserves necessary to emerge from deep in the soil.
Care should be taken when planting into dry soils. A light rain on seed planted too shallow may cause the seed to germinate but not provide enough moisture to maintain emergence. Heavy rains on seed planted to deep may prevent the seed from emerging due to soil crusting and compaction. In this case seed may lack the necessary vigor to emerge through the tight crust.
Table 12. Relation of planting depth and seedling emergence*
Depth of planting (in.)
|
Emergence (% of total planted)
|
Depth of root system below soil surface (in.)
|
||
Normal**
|
Abnormal***
|
Non-emerged
|
||
1
|
93
|
2
|
4
|
0.8
|
2
|
76
|
15
|
8
|
0.9
|
3
|
31
|
52
|
15
|
1.3
|
4
|
4
|
45
|
49
|
1.5
|
5
|
0
|
32
|
67
|
—
|
*Source: S.C. Chambers. 1963.
**Normal: Coleoptile emerges from the soil before the appearance of the first leaf.
***Abnormal: Coleoptile fails to appear, and first leaf emerges from the soil.
Row Width
Yields of more than 100 bushels/acre have been achieved with 7-in. row spacings. When wheat is planted in 4-in.-wide rows it generally produces 5 percent to 10 percent more grain than wheat planted in 7-in.-wide rows. This suggests that row width should be a consideration when it comes to buying a new drill, but is not a large enough factor alone to mandate the purchase of new equipment.
WEED MANAGEMENT
Weeds affect small grain production in many ways. They compete with the crop for light, water, nutrients and physical space, thereby reducing yields and quality. Weed chaff and seed contaminate harvested grain, resulting in dockage, and the presence of green weeds reduces harvest efficiency and increases grain moisture content.
It is estimated that the total loss from weeds in wheat is more than $29 million in the southeastern United States and more than $850 million nationally.
Weeds in Small Grains: Biology, Distribution and Resistance
Several grass and broadleaf species are common in small grain fields throughout Georgia and the Southeast (Table 13).
Table 13. The 10 most common and troublesome weeds infesting small grains in Georgia, ranked in order of importance
Common name | Scientific name |
Commonness
|
Troublesomeness
|
Wild radish | Raphanus raphanistrum |
1
|
1
|
Italian ryegrass | Lolium multiflorum |
2
|
2
|
Wild onion/garlic | Allium vineale, A. canadensis |
3
|
3
|
Henbit | Lamium amplexicaule |
4
|
5
|
Curly dock | Rumex crispus |
5
|
4
|
Cutleaf evening primrose | Oenothera lacunata |
6
|
6
|
Common vetch | Vicia sativa |
7
|
7
|
Swinecress | Coronpus didymus |
8
|
8
|
Little barley | Hordeum pusillum |
9
|
9
|
Common lambsquarters | Chenopodium album |
10
|
10
|
Source: Adapted from Weed Survey-Southern States in Proceedings, Southern Weed Science Society, 1994, vol. 47:273-299.
Broadleaf (dicot) Weeds
Eight of the 10 species are winter annual broadleaf (dicot) weeds. Brassica species, like wild radish, wild mustard, wild turnip (bird's rape mustard), swinecress, Virginia pepperweed and shepherd's purse, are the most common broadleaf weeds in small grains in Georgia. Perennial weeds such as curly dock, common mullein and thistles are troublesome, especially in minimum tilled areas. Wild onion and/or garlic are generally less competitive with small grains than wild radish, but they pose grain quality and dockage problems in harvested grain.
Like wheat, most of these weeds germinate, emerge, and grow vegetatively during the fall. Limited growth occurs during winter. Then in late winter or early spring rapid growth resumes, and the plant transitions into reproductive development. Light, water and nutrients are critical during the reproductive phase. Therefore, competition by weeds during this period is very damaging to crop growth and yield. Without good weed control, tillering is reduced, which limits the number of seed heads. Weed competition can reduce head size and grain weight.
Wild radish is generally regarded as a winter annual species, but this weed emerges almost year-around. It is important to plant small grains into a stale or clean seedbed in order to eliminate early-season competition from radish that emerges ahead of cereal planting. Depleting the soil seed bank of wild radish seed will require a year-around effort to prevent seed production. It is noteworthy that the imidazolinone herbicides (Scepter, Pursuit and Cadre) are very active on radish. Because of the persistence of these herbicides, fall suppression of wild radish may result from summer applications of these herbicides to soybeans or peanuts.
Grasses
Several grasses are common to small grain fields in Georgia: Italian (annual) ryegrass, cheat and little barley. Italian ryegrass is the most important grass found in small grain fields. It is extremely competitive with the small grains, causing lower yields, reduced quality, decreased harvest efficiency, and contamination problems. Cheat and little barley are generally not as competitive as ryegrass and infest limited acreage.
Although the 1994 survey shows that Italian (annual) ryegrass is the second most common and troublesome weed in grains in Georgia, it has now become the most difficult-to-control weed for most Georgia farmers, primarily because of control failures with diclofop-methyl (Hoelon). Several factors contribute to control failures (see the Italian ryegrass control section), but one of the more important factors has been the development of Hoelon resistant Italian ryegrass. Italian ryegrass emerges before or with winter cereals and grows very rapidly, competing vigorously with winter cereal grains for nutrients, light, and water. More effective management of Italian ryegrass is essential if Georgia farmers are to grow winter small grains at a profit.
Weed Identification
Accurate weed identification is essential for selecting the most effective and economical weed control tactic. Distinguishing characteristics for some of the most common weeds are shown in Table 14.
Table 14. Weeds common in Georgia winter grain fields
Common name | Description |
Annual ryegrass | Bunchgrass; leaves dark, shiny green; auricles present (clasping structures where leaf connects with the stem). |
Wild onion | Flat, not hollow onion-like leaves. |
Wild garlic | Hollow, tube-like onion-like leaves. |
Wild radish | Light yellow flowers; mature leaves deeply dissected (segmented); immature leaves densely hairy; heart-shaped cotyledons with purple hypocotyl; lower portion of stem with stiff downward pointing hairs. |
Wild mustard | Dark yellow flowers; leaves slightly dissected and not attached to the stem with clasping appendages; heart-shaped cotyledons but with sparse or no hairs with green hypocotyl. |
Wild turnip | Similar to wild mustard; dark yellow flowers; uppermost leaves clasp the stem. |
Curly dock | Perennial, forming a rosette of leaves often exceeding 12 in. in diameter; leaf margins often curly or wavy, tapering at the base. |
Henbit | Stems 4-sided (square) with purple cast; leaves opposite with long internodes; small purple flowers are formed in whorls arising from the base of the leaves. |
Vetch | Winter annual legume with compound leaves containing 3-9 pairs of leaflets; climbing plant with tendrils present at the ends of the stems. |
Cutleaf evening primrose | Leaves form rosette with notched/lobed margins; distinctive white midrib of leaves. |
Swinecress | Leaves highly serrated, formed in a rosette; strong odor when the leaves are crushed. |
Carolina geranium | Long leaf stems (petioles), densely hairy; leaves roundish and deeply dissected. |
Little barley | Bunchgrass; light green leaves; membranous ligule; seedheads similar in appearance to barley or wheat only much smaller with short, stiff awns. |
Management and Control
Cultural Weed Control
Cultural weed control plays a major role in reducing weed pressure in small grains. Purchasing certified seed ensures quality, weed-free seed and reduces the potential for the introduction of new weed species. In Europe, corn-cockle (Agrostemma githago) infestations were reduced by over 91 percent through the use of certified wheat seed. The planting of bin-run wheat and rye seed has probably contributed to the spread of wild radish and Italian ryegrass throughout the state. Proper seedbed preparation, fertility, insect and disease control will promote a healthy crop, allowing the crop to shade and out-compete many weeds. Selection of well-adapted varieties and adhering to optimum planting dates will also contribute to a healthy and vigorous grain crop. Cleaning combines will prevent moving weed seed from one field to another.
Grazing small grains that will be harvested for grain may change weed control practices. Cattle often selectively graze on small grain forage and leave the less palatable weeds. Selective grazing may diminish the competitiveness of the crop relative to the weeds so that weeds that are normally considered relatively unimportant may become well-established and reduce grain yields once grazing ceases.
Rotation can be an important practice for weed control. It is often associated with changes in tillage, planting date, crop competition, and herbicide use, all of which can improve long-term weed management. Incorporating canola or a winter fallow into a continuous small grain production sequence can improve Italian ryegrass control. With a winter fallow one can presumably prevent ryegrass seed production and possibly reduce ryegrass seed reserves in the soil. Similarly, canola producers have two options for ryegrass control, preplant applications of trifluralin (Treflan) and post-emergence applications of sethoxydim (Poast) or quizalofop (Assure). Rotations with other winter crops may provide similar benefits to small grain growers.
Mechanical Weed Control
Because between-row cultivation is not possible with drilled small grains, preplant tillage provides the only opportunity for cultivation. Preplant tillage can be used in combination with herbicides to accomplish a "near stale seedbed" condition. Preplant tillage will also minimize problems with perennial species like curly dock. Mowing is not a viable option because most weeds do not bolt until the small grain has begun to elongate. However, mowing may be an acceptable alternative in small grains used for grazing.
Chemical Weed Control
Numerous herbicides are available for weed control in small grains. To maximize performance weeds must be properly identified so that the most effective herbicide can be applied in a timely fashion. Recognition of the following crop stages is critical to effective herbicide use in small grains: seedling stage, tiller stage, jointing stage, and dough stage. Tillers are secondary (axillary) shoots that arise from the base of the plant. With proper fertility management and growing conditions, most plants will form four or more tillers. Once tillered, the plant reaches a resting stage called full tiller, where vernalization is required to cause stem elongation and head formation. This stage of elongation is called jointing. Dough stage occurs as the grain begins to develop, fill and harden. These growth stages are used to determine herbicide application timing.
Broadleaf Weed Control - Phenoxy Herbicides
The phenoxy herbicides, 2,4-D and MCPA, are used for broadleaf weed control in small grains. They have little effect on grasses. They are growth regulators that cause uncontrolled growth in susceptible plants. Most small grains have good tolerance to the phenoxy herbicides, but certain guidelines with respect to crop developmental stage should be followed to minimize injury.
- Phenoxy herbicides should be applied to grain crops that have at least 3 tillers. Applications made before this stage will cause a "rat-tail" or "onion-leaf" effect, where the leaf does not form and unfurl properly. Tiller number can be reduced, the plant may be stunted, and maturity can be delayed.
- Phenoxy herbicides should be applied before jointing. Once the stem begins to elongate (jointing stage) cereal grains can be damaged, resulting in kernel damage and malformed seed heads.
2,4-D is the most active of the phenoxy herbicides. It gives excellent wild radish control, but the potential for crop injury is greater than that with MCPA, especially if it is applied too early. It can be applied from the 3 to 4 tiller stage until jointing begins at rates from 0.5 to 1.5 pints/acre (0.25 to 0.75 lbs-ai) plus a surfactant at 0.25 percent v/v. It is typically more effective on larger plants (3 in. or larger) than is MCPA, but the potential for injury is greater than with MCPA. Application timing is critical to achieve weed control and crop safety. 2,4-D should not be applied with liquid nitrogen! Excessive crop injury is likely to result. The optimum timing for application of nitrogen and 2,4-D typically differs. Mid- to late-December applications of 2,4-D are optimum for wild radish control, but late January to mid-February is optimum for nitrogen fertilization. Therefore, applying the two together in a single pass usually results in either the nitrogen being applied too early or the 2,4-D being applied too late.
MCPA is another phenoxy herbicide labeled for use in small grains. It is less injurious to wheat, but is also less effective on larger weeds. It should be applied very early to small wild radish at rates from 0.5 to 1.5 pints/acre (0.25 to 0.75 lbs-ai) plus a surfactant at 0.25 percent v/v. MCPA can be applied as soon a tiller begins up until full tiller.
Broadleaf Weed Control - Sulfonylurea Herbicides
Three sulfonylurea herbicides are currently registered for weed control in small grains in Georgia. These materials inhibit the production of three essential amino acids in susceptible plants, causing stunting and slow death over a period of 10 days to 3 weeks. With respect to crop safety, sulfonylurea herbicides have a wider range of application timing as compared to the phenoxy herbicides. They can be applied before and after wheat is fully tillered. However, the susceptibility of weeds declines rapidly with increases in age and size of weeds. Rotational crop restrictions exist for most sulfonylurea herbicides. Therefore, check the label regarding rotational restrictions. To maximize weed control:
- Do not apply sulfonylurea herbicides to stressed plants (i.e., in abnormally cold, wet or dry weather). Efficacy is greatest in rapidly growing susceptible plants.
- Apply to small weeds and use a nonionic surfactant to ensure adequate coverage.
Express (tribenuron) will provide good control of wild radish but should be applied early for best control. Applied at 1/3 oz product/acre from the two-leaf stage through the third node of joint. Express, applied alone, is marginally effective on large wild radish. Therefore, Express should be tank-mixed with MCPA. When applying with MCPA, follow the MCPA guidelines with respect to timing of applications. This tank-mix is a good, safe alternative to early applications of 2,4-D. Express is only labeled for use in wheat or barley. Do not use on rye or oats.
Harmony Extra (thifensulfuron + tribenuron) will provide good control of wild radish and many broadleaf weeds in wheat. It can be applied from the two-leaf stage through the third node of joint at rates from 0.4 to 0.6 oz product/acre plus surfactant (0.25 percent v/v). Harmony Extra is the product of choice for vetch control. However, it must be applied at the higher rate to non-stressed, actively growing vetch for maximum control. Harmony Extra also can be tank-mixed with MCPA or 2,4-D. Harmony Extra is registered for use on wheat, oats, and barley.
Peak (prosulfuron) is registered for use on wheat, rye, barley, oats and triticale. It has good activity on wild radish, cutleaf evening primrose and wild onion/garlic, and partial suppression of henbit. The rate of 0.5 oz/acre is recommended with the addition of a non-ionic surfactant at 0.25 percent v/v. It can be mixed with 2,4-D or MCPA or liquid nitrogen. It should be applied after the third leaf has emerged and prior to early joint.
Broadleaf Weed Control - Buctril (bromoxynil)
Buctril (bromoxynil) is a contact herbicide that is sold alone or in combination with other materials.
It is safe to small grains and can be very effective for early-season control of wild radish. For best results, apply to small weeds having no more than four true leaves. Buctril is often premixed or tank-mixed with MCPA (Bronate) for improved control of weeds such as mustard and wild radish.
Broadleaf Weed Control - Sencor (metribuzin)
Sencor can provide good control of Italian ryegrass, wild radish, and other broadleaf weeds in wheat. See the section on Sencor in the following section on Italian ryegrass control.
Italian Ryegrass Control - Hoelon (diclofop-methyl)
Hoelon is registered for use in wheat and barley. Maximum activity will be achieved if Hoelon is applied to two- to four-leaf Italian ryegrass. Effective control can be achieved at this stage with as little as 11/3 pints of product per acre. Once ryegrass has progressed beyond the four-leaf stage, use higher rates up to 22/3 pints/acre. Hoelon can be used pre-emergence in wheat. However, it should be used at the higher labeled rates. Several factors affect the use and performance of Hoelon on Italian ryegrass:
- Hoelon-resistant Italian ryegrass has been identified in Georgia. On these sites, Hoelon will not control ryegrass and its continued use will only make the situation worse.
- Early applications (i.e., December) are much more effective than later applications to fully tillered and hardened ryegrass.
- Environmental stress (i.e., drought or cold temperatures) will significantly reduce Hoelon activity on ryegrass.
- Using crop oil concentrate (COC) at 1 quart/acre will enhance ryegrass control with Hoelon compared with the use of nonionic surfactant.
- Wheat injury, primarily temporary yellowing, has been observed in wheat that was treated with organophosphate insecticides like Di-Syston.
- Do not tank mix Hoelon with other herbicides or liquid nitrogen.
- Do not apply to oats.
Italian Ryegrass Control - Sencor (metribuzin)
Sencor is labeled for use on both wheat and barley in Georgia. It can be used postemergence to wheat or barley after the third tiller provided wheat is planted prior to November 15 and December 1 in the Piedmont and Coastal Plain regions of the state, respectively. Rates are dependent on soil type and crop stage of growth. Therefore, consult the label. Sencor is labeled for control of wild radish. Italian ryegrass does not appear on the label. However, control can be good depending on environmental conditions.
The following precautions should be observed when using Sencor on small grains:
- Do not apply to oats.
- Some cultivars of wheat and barley are sensitive to Sencor. Do not plant sensitive cultivars if you plan to use metribuzin. Consult with your local county extension office and/or the Sencor label for a current listing of tolerant versus sensitive wheat and barley cultivars.
- Carefully read the label to select the proper rate based in soil type and stage of wheat/barley growth.
- Early applications (i.e., four-leaf stage of wheat) are typically more effective on Italian ryegrass than late applications.
Weed Control in Rye and Oats
Relatively few herbicides are labeled for weed control in rye and oats. However, the phenoxy herbicides (2,4-D, MCPA, etc.) can be used effectively for broadleaf weed control in rye and oats. Control in these small grains is limited to the phenoxy materials and Peak. The application timings are the same for these crops: three to four tillers to full tiller with rates from 0.5 to 1.5 pints/acre. Oats are generally more sensitive than rye. There is nothing labeled for annual ryegrass control. There are 7- and 14-day grazing restrictions for MCPA and 2,4-D, respectively, and a 30-day grazing restriction for Peak.
Liquid Nitrogen and Herbicide Tank-Mixes
Many farmers will apply liquid nitrogen and herbicides in a tank-mix for a single application to cut costs. Tank-mixing is a practical and cost-effective, but it is often a compromise. If herbicides and nitrogen are to be mixed, make sure that application occurs within the optimum application window for both materials. Making applications early may increase weed control, but it may increase crop injury and risk nitrogen deficiency during grain fill. Delaying applications for maximum fertility benefit increases the chance of crop injury with phenoxy herbicides and reduces weed control. Wheat yellowing will often be observed when phenoxy herbicides are applied with liquid nitrogen. This can be minimized by not using surfactants in these mixes unless the herbicide label specifically calls for it.
Herbicide Carryover
Residues that remain from herbicides applied to preceding crops like cotton, peanuts and corn can cause damage to small grains. Although this is not a serious problem, injury is often observed in some fields throughout the state, especially in early-planted grains. Oats generally are more sensitive than wheat, rye or barley. Injury has been observed with Zorial, which has an 18-month rotation restriction for small grains. Also, injury can occur, especially with oats, where Karmex is used as a late-season layby treatment in cotton. Plan your rotations and consult herbicide labels for rotational restrictions.
The herbicides mentioned in this chapter were registered for use in Georgia at the time the chapter was prepared. However, always consult and follow current label directions when using pesticides.
DISEASES OF WHEAT
Diagnosis of Disease Problems
Wheat may be affected by a number of parasitic diseases and nonparasitic problems. Parasitic diseases are caused by bacteria, fungi, viruses and nematodes. Nonparasitic problems may be caused by weather extremes, nutritional imbalances, pesticide injury and many other factors.
The first step in diagnosing a problem is to look at the entire field. What is the distribution of affected plants? A general yellowing, stunting, or other symptoms over the entire field is not likely to be a disease. Look to see if the problem is in some type of pattern associated with differences in terrain, soil type or cultural practice that may account for differences in growth. A soil fertility problem often shows up as yellow to light green streaks throughout all or part of the field. Fertility problems may require soil and tissue samples for accurate diagnosis. Distribution of the problem in the field may also suggest the type of disease. For example, a circular to oval pattern is characteristic of a root disease because the disease usually moves only a short distance in one growing season.
The next step in diagnosis is to look for more specific symptoms. Stunted plants or discolored leaves suggest that something could be wrong with the roots. Blackened or discolored areas on the roots strongly suggest a root disease.
Spots on the leaves, stems or heads suggest some type of parasitic disease. You can often find signs of a fungus or bacterium in these lesions which are diagnostic for the disease. These may be bacterial ooze, fungal spores (reproductive units), fruiting bodies containing spores, or the threadlike growth of a fungus.
Accurate diagnosis is important so that the correct control measures can be followed. Accurate identification of the disease may require that a sample be sent to a trained pathologist. Samples should be taken from plants where the symptoms are actively developing. As disease progresses, the amount of dead tissue increases and the diagnostic symptoms are more difficult to see. Be sure you include enough plant material for accurate diagnosis. Do not store samples for prolonged periods in plastic bags. Samples in plastic bags may get overheated if placed in sunlight or secondary molds can grow quickly which obscure the symptoms. Plants that are dry are still useful for diagnosis or isolation of pathogenic organisms. Take the sample to your county Extension agent. Be able to give as much information as possible on cropping history, variety, fertility and pesticide applications, and distribution in the field. Your county Extension agent may send the sample to the Extension Plant Disease Clinic to confirm diagnosis. Photos of disease symptoms can be submitted by Extension agents in Georgia via the Internet using the Distance Diagnostics through Digital Imaging system (accessed at https://www.dddi.org/uga/).
Leaf and Head Diseases
Leaf diseases are especially prevalent in the Southeast because the climate is humid, with frequent rains during the period from jointing to maturity. Prolonged periods of moisture allow disease-causing fungi and bacteria to multiply and spread from diseased to healthy plants. Three foliar diseases caused by fungi are of major importance each year. These are powdery mildew, leaf and glume blotch, and leaf rust. Yield losses up to 30 percent for each disease have been documented in seasons when environmental conditions were favorable. Even in seasons when yield losses are relatively low, test weight may be reduced sufficiently to make the grain unacceptable for milling and baking. Several other leaf diseases may be important in some seasons or on certain varieties.
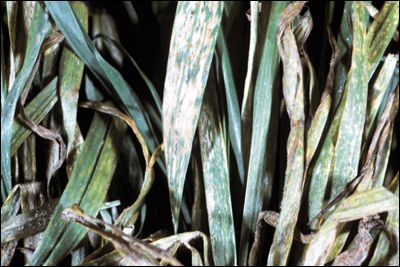
Powdery Mildew
Powdery mildew, caused by Blumeria graminis f. sp. tritici (syn. Erysiphe graminis) produces white to gray cottony fungal growth on the upper leaf surface (Figure 6). Small black fungal fruiting bodies may be seen in the gray fungal tissue later in the season. Powdery mildew is common on the lower leaves when plants begin to joint. It is usually the first leaf disease to appear in the spring, because it is favored by temperatures between 50° and 70°F. The disease usually declines after flowering when temperatures rise above 75°F.
However, it can cause severe blighting of the upper leaves and can attack the head of susceptible varieties. Powdery mildew can infect plants in the fall and survive in the invaded tissue during the winter. Early infection can result in reduced tillering of susceptible varieties. Thus, infective spores may come from earlier infections within the field or from fields farther away.
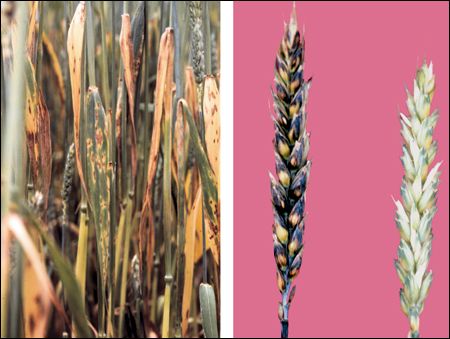
Leaf and Glume Blotch
Leaf and glume blotch or Stagonospora blotch, caused by the fungus Stagonospora nodorum (syn. Septoria nodorum), first attacks the lower leaves and progresses up the plant (Figure 7). The lesions are at first circular to elliptical, brown in the center with a narrow yellow margin. Usually many infected areas join together making the lesions irregular in shape. The lesion centers often become light brown with numerous dark brown circular fruiting bodies called pycnidia embedded in the dead tissues. Pycnidia produce spores which are spread by splashing rain to upper leaves and the head or to nearby plants. These spores immediately invade leaves initiating a new disease cycle. The leaf blotch phase of the disease first appears at jointing stage but is most noticeable after flowering when spots develop on the upper leaves. Temperatures from 60° to 75°F are optimum for disease development. Frequent rains allow S. nodorum to spread rapidly and initiate many new lesions. Because S. nodorum spores are not disseminated more than a few feet from infected plants, the source of infection is within the field.
The fungus also invades the glumes (chaff) and the disease is often referred to as glume blotch (Figure 7). Symptoms on glumes are typically oval, usually starting on the upper half. Lesions are tan to dark brown and contain the flask-shaped pycnidia, especially after a wet period. Diseased leaves and glumes lose their chlorophyll prematurely which prevents normal grain filling. Kernels are shriveled and yield, test weight and 1,000 kernel weight are reduced. Dark "sooty" molds may develop on the dead tissue of blighted heads and healthy ripe heads if rainy weather occurs. In these instances, the 'sooty' molds are sometimes mistaken for glume blotch. S. nodorum is also carried in seed as a result of head infection and thus both seed and infected plant debris serve as sources of infection for the following season.
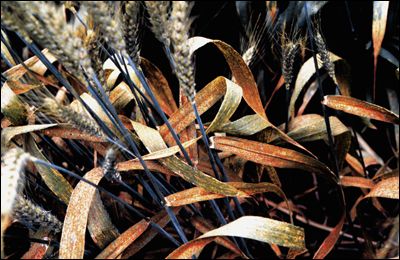
Leaf Rust
Leaf rust, caused by the fungus Puccinia recondita, is often the most destructive foliar disease of wheat in the Southeast (Figure 8). Small pustules about 1/16 in. in diameter with flaky red spores develop rapidly on the upper leaves. Pustules may be found early in the growing season on lower leaves, but they often appear in large numbers on the upper leaves after flowering. This is because the disease is favored by temperatures from 60° to 80°F, and large numbers of spores are disseminated long distances by air from fields farther to the south and west. A single spore can invade a leaf and produce a pustule with thousands of new spores 8 to 10 days later. This permits the disease to spread quickly and cause extensive damage within a short time.
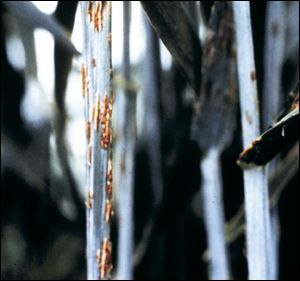
Stem Rust
Stem rust (Puccinia graminis f. sp. tritici) is a potentially important disease because, like leaf rust, it can cause significant losses in a short time. Pustules are dark red and two to three times larger than those of leaf rust (Figure 9). They are found most commonly on the stem and leaf sheaths. Broken portions of the leaf epidermis are evident around the pustules. Stem rust only occurs following mild winters and when the spring season is wet. It occurs less frequently because the fungus does not survive as well as leaf rust during the winter in the Southeast. Susceptible varieties are not recommended for planting.
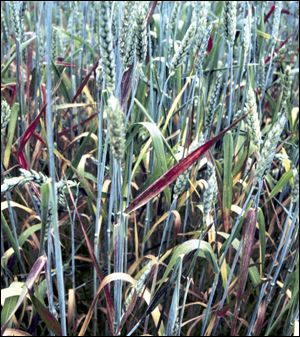
Barley Yellow Dwarf
Barley yellow dwarf virus is transmitted to wheat by several species of aphids from grassy weeds or oats and barley (Figure 10). Barley yellow dwarf (BYD) is favored by early planting and mild winters which extend the active period of aphid vectors. Symptoms of barley yellow dwarf are quite variable. If infection occurs in the autumn, plants are often stunted. Severe stunting is more common in barley and oats. Affected wheat plants are reduced in size and heads have fewer seeds than healthy plants. Symptoms in wheat vary from yellowing to reddening or purpling of the upper leaves beginning at the leaf tips and extending backward toward the base. Symptom color varies with the variety and may be similar to those caused by nutrient imbalances.
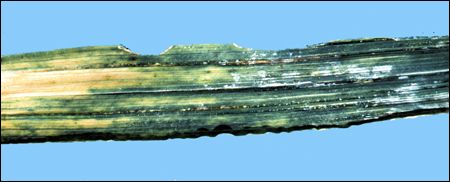
Bacterial Stripe or Black Chaff
Bacterial stripe or black chaff, caused by the bacterium Xanthomonas campestris pv. translucens, has been found more frequently in recent years in the southern United States (Figure 11). It has not been a significant problem in Georgia. Symptoms are very long, narrow stripes along the leaf veins. The stripes are often water-soaked or oily looking during damp conditions and yellow droplets containing bacteria may be present on the leaf surface. The glumes may also exhibit brown blotching or striping. Bacterial stripe is often common on triticale, and this crop may be a source for infection of wheat (see "Triticale Pest Management" section).
Fusarium Head Blight
Fusarium head blight or scab is caused by the fungus Fusarium graminearum, which also causes an ear and stalk rot on corn. Because wheat seldom follows corn in most rotations in the region, carryover of the fungus in the field is greatly reduced. Scab may also increase if wheat is planted after sorghum. Scab is potentially a problem in wheat when there is prolonged wet weather during flowering. The affected portion of the head dies prematurely and turns white. Seed either do not form or are severely shriveled, have a pinkish-white (scabby) color, and have a very low germination.
An additional problem is that the fungus produces a compound in the grain that is toxic to livestock and man. The incidence of scab has been very low in Georgia, but it is potentially a problem if environmental conditions are favorable.
Seedling Diseases
Seed rots and seedling diseases can sometimes cause stand problems in wheat and other small grains. Symptoms of these diseases are variable, ranging from a rotting of the seed and seedling before emergence to a root and lower stem rot after emergence. Seedling diseases are more common in warmer soils for small grains and thus are more serious in early plantings. Several different fungi cause these stand problems.
They include Rhizoctonia, Pythium, Helminthosporium and Fusarium. Pythium and Rhizoctonia are soilborne. Helminthosporium and Fusarium survive in the soil but are common on seed also. Seed treatment fungicides (Table 15) used in combination with correct planting time and depth of planting help to control soilborne seedling diseases.
Table 15. Fungicide seed treatments for small grains (wheat, oats, barley, rye)
Fungicide | Crop | Rate/100 lb seed | Remarks |
Benomyl | All | 1.7-3.3 oz | Grazing not permitted |
Captan-maneb | All | 2-4 oz | Can be used for drillbox treatment |
Captan | All | 2-4 oz | Can be used for drillbox treatment |
Carboxin-captan |
Wheat, oats, barley | 4 oz | Controls loose smut but not as drillbox treatment |
Carboxin-thiram (Vitavax 200) (RTU-Vitavax-thiram) |
Wheat, oats, barley | 4 oz 5-6.8 fl oz |
Controls loose smut Commercial or drillbox application |
Difenoconazole-metalaxyl (Dividend XL RTA) |
Wheat | 5-10 oz | Controls loose smut, Pythium damping-off Commercial or drillbox application |
(Dividend/Apron) | Wheat | 0.2 oz | Controls loose smut, Pythium damping-off Commercial or drillbox application |
Mancozeb (Penncozeb 80WP)* (Dithane m-45) |
All | See label | Drillbox only |
Metalaxyl (Allegiance-FL) | All | 0.375- 0.75 fl. oz |
Controls Pythium damping-off |
(Apron 50 W) | All | 0.5-1 oz | Controls Pythium damping-off |
( Apron XL) | All | 0.32-0.64 oz | Controls Pythium damping-off |
Tebuconazole-thiram (Raxil-thiram) |
Wheat, oats, barley | 3.5-4.6 fl. oz | Controls loose smut |
* Examples of formulations in parentheses. Read all labels carefully for use restrictions and most current recommendations.
Seedborne Diseases
Certain fungi that cause diseases of more mature plants may be seedborne. These include Stagonospora nodorum and smut fungi.
Loose Smut
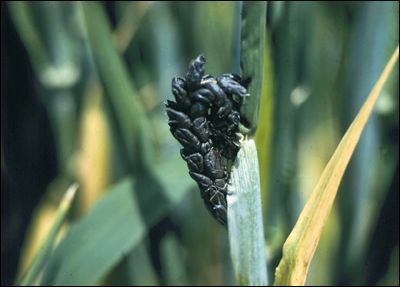
The loose smut fungus (Ustilago tritici) infects the seed, but symptoms are not seen until the following year when the grain head emerges (Figure 12). The fungus remains dormant until the seed germinates. As the plant grows, the fungus grows within it. When the grain head emerges, the flowers are replaced by masses of smut spores. These spores are wind-blown to healthy flowers where infection takes place and the cycle is repeated.
Common or Stinking Smut
This disease occurs infrequently in the Southeast, but it can be severe. Two related fungi, Tilletia tritici and T. laevis, produce black smut spores, which attach to seed during harvest. When the seed germinates, the fungi invade the seedling and grow systemically like loose smut. However, heads develop normally at first. As seed forms, only portions of the seed coat remain. The remainder of the tissue is converted to round "bunt balls" filled with masses of dark spores. This mass of spores contains trimethylamine, a compound that gives the grain the odor of dead fish. These bunt balls break during harvest and contaminate healthy seed with spores. The smut spores can survive in soil in arid regions, but they do not survive in soil in the humid Southeast. Stinking smut only becomes a problem when seed is saved for several years which allows the fungi to build up and when no seed treatment fungicide that controls smuts is used. Use of certified seed prevents the establishment of stinking smut.
Karnal Bunt or Partial Bunt and Ryegrass Bunt
Prior to 1996, Karnal bunt, caused by the fungus Tilletia indica, was not reported in the United States. It was introduced into Mexico about 1970, probably on seed from Asia. Seed with a few bunted kernels were found in Arizona in 1996. Bunt spores are carried on seed and can survive for up to 5 years in soil. The spores do not infect seed or seedlings like other smuts. The dark smut spores germinate on the soil surface when wheat is heading. They produce secondary spores, which are carried by air to the head. If infection occurs during or shortly after flowering, the entire seed is bunted. Later infection results in partial bunting of the seed. Sometimes only pinpoint infections may occur on the seed. Because individual seeds are infected, there may only be one or a few infected kernels per plant.
Suspected Karnal bunt spores were found on wheat seed in the Southeast in 1996, but no bunted kernels have been found. In the Southeast, in Oregon, and in several other countries, spores that resemble the Karnal bunt fungus have been associated with annual and perennial ryegrass seed. The spores found on wheat from the Southeast were from areas where ryegrass is a common weed problem in wheat. These spores of ryegrass bunt are deposited on wheat seed during harvest. Ryegrass bunt has recently been described as a new species, Tilletia walkerii, which is distinct from Karnal bunt. Therefore, Karnal bunt has not been found in the Southeast. Karnal bunt seldom causes significant yield loss, and it can be controlled by the same methods used to control stinking smut and loose smut. Currently Karnal bunt remains a quarantined disease in the U.S. However, it poses no threat to the U.S. wheat crop and efforts are being made to deregulate it.
Soilborne Diseases
Several diseases affect below-ground plant parts of wheat. Unlike many leaf diseases these do not spread over long distances and thus are often found as small areas of diseased plants in fields. They also do not produce many cycles of propagules during the growing season. Because of their limited spread, it may take several consecutive years of wheat production for a soilborne disease to become severe in a field. Soilborne diseases important on wheat in the Southeast are take-all, Pythium root rot, soilborne wheat mosaic and wheat spindle streak mosaic. Several other soilborne diseases may be found in some seasons.
Take-all
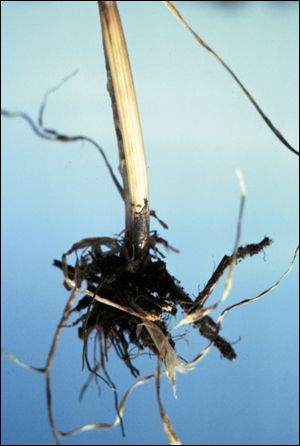
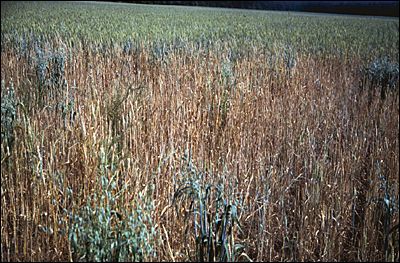
Take-all, caused by the fungus Gaeumannomyces graminis var. tritici, attacks the roots and basal portion of the stem of wheat and other small grains (Figure 13). Take-all is usually observed as scattered circular to oval areas of stunted plants which mature early, resulting in whiteheads which have no or shriveled seed (Figure 14). Whiteheads caused by take-all occur mostly in May (about milk stage) long after any chance of late frost. Take-all diseased plants tiller poorly and are easily pulled from the soil because of a rotted root system. The roots are blackened and brittle and if the lower leaf sheath is peeled back, the basal portion of the stem may be black due to the growth of the fungus. These dead plants may become discolored by harvest time as a result of the growth of dark "sooty" molds on aboveground plant parts. These areas may be excessively weedy due to the poor growth of the wheat. The fungus may also colonize a sizeable portion of the root systems of plants in fields that have no whitehead symptoms.
Surveys indicate approximately 20 percent of wheat fields in Georgia have stunted plants or plants with whiteheads due to take-all. Take-all occurs most frequently in fields having three or more consecutive years of wheat. Triticale, barley, and rye are less susceptible than wheat. Oats are resistant to the disease. Yield losses of 66 percent for wheat, 50 percent for triticale, and 34 percent for barley have been documented.
The fungus infects plants throughout the growing season, but plants infected earlier sustain greater losses. The fungus survives on residue of plants infected during the previous season. Movement of crop debris within fields and between fields should be minimized. In a study in Georgia, crop rotation without wheat for one year reduced disease from 57 percent to 8 percent whiteheads. Two years though may be needed to allow residue to decompose completely so the fungus dies. A one year rotation with canola can also reduce severe take-all to insignificant levels. A summer rotation with sorghum greatly reduces disease compared to a fallow or soybean summer rotation. The take-all fungus is associated with various grassy weeds and volunteer wheat. These infected plants may be important in survival of the fungus in the absence of a susceptible crop. Soil pH above 6.0, and cool wet soils (54° to 61°F) favor disease, whereas a dry spring can limit the development and expression of whitehead symptoms.
Pythium Root Rot
Pythium fungi are found in all soils and attack a wide range of plants. Diseased roots usually develop soft tan-brown areas. Pythium root rot may cause slight stunting of the plants and reduced tillering. Pythium species reduce yields considerably, especially in reduced tillage systems. Research in Georgia has found that Pythium species are the most common group of pathogenic fungi isolated from wheat seedlings. Within three weeks after planting, 50 percent or more of the wheat plants have roots infected with Pythium species. However, the yield losses associated with this group of fungi on wheat are still unknown. Disease is favored by compacted, wet soils. Early planting when soil temperature is high favors Pythium species, which kill seeds and germinating seedlings. These are most prevalent in the coastal plain.
Soilborne Viruses
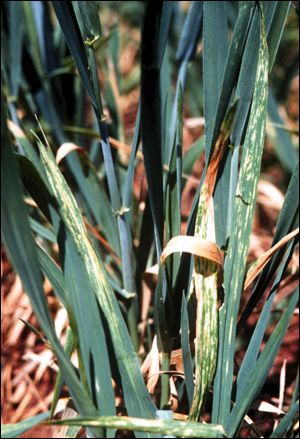
Soilborne wheat mosaic virus has been known in the Southeast for many years. It seldom causes much damage because most varieties are resistant. Another soilborne virus, wheat spindle streak mosaic virus (WSSMV), was identified for the first time in the Southeast in 1984 at Plains, Georgia (Figure 15). Since then it has been identified throughout Georgia and nearby states. Both viruses are transmitted to wheat by a fungus (Polymyxa graminis) that invades the roots. The fungus causes no damage to the roots but carries these viruses with it. The fungus invades the plant during cool, wet weather (40° to 50°F) in the fall and early winter.
Plants severely diseased by WSSMV are already stunted and yellowed by mid-to-late February in central Georgia. The yellowing of leaves appears as many very narrow (1/16 in. or less) stripes. Tillering is greatly reduced and diseased plants mature later than normal. Yield losses from 30 to 67 percent have been documented on susceptible cultivars. The disease appeared because susceptible varieties were planted several consecutive years in the same fields. Precise information is not available, but crop rotation for one or more years is needed to reduce the virus population in the soil. Selection of a resistant variety is critical for control.
Other Soilborne Diseases
Numerous other root diseases occur on wheat, but are of minor importance in the Southeast. These include common root rot, caused by Helminthosporium sativum and Fusarium graminearum, and Rhizoctonia root rot and sharp eyespot, caused by Rhizoctonia solani and closely related fungi. H. sativum causes dark brown lesions on roots and especially the subcrown internode. F. graminearum causes a dry browning of the roots and base of the stem; these plant parts may be reddish due to fungal growth. Common root rot is favored by drought and temperatures from 70° to 85°F.
Rhizoctonia and related fungi attack the roots and stems of wheat. Rhizoctonia cerealis also causes eyespot lesions on lower leaf sheaths and the base of the stem. The lens-shaped lesions have dark brown borders and tan centers.
Nematodes
Several species of plant parasitic nematodes attack wheat in the Southeast. Root-knot, caused by Meloidogyne spp., is the most common disease. Unless wheat is grown continuously for several years, it is seldom a problem.
Integrated Disease Management
Diseases can be managed by a number of methods incorporating principles of IPM (Table 16). A fully integrated system can reduce input costs and reduce pesticide use. Discussion of these methods follows:
Seed Quality and Treatment
Seed quality is important for several wheat diseases that may be transmitted by infected seed. Seed treatment with carboxin, triadimenol, and difenoconazole provides nearly complete control of loose smut and other smuts. Stagonospora nodorum is transmitted by seed, but the fungus also survives on wheat straw and weeds. Seed treatment will help control leaf and glume blotch when combined with crop rotation. Seed treatment is important in reducing seedling diseases caused by soil fungi.
Wheat and other small grain seed can be treated in one of three ways: commercial treatment, a homemade rotary treater or drill box treatment. Commercial treatment is the most effective. Use of a homemade treater is slow but nearly as effective as commercial treatment. To use this method, fill a 30 gallon barrel about half full with seed, add the recommended amount of fungicide, and rotate 25 times. Seed can be treated several weeks before planting and stored until needed. Drill box treatment is the least effective seed treatment method. To use the drill box method, fill the grain drill about half full, add the required fungicide and stir into the seed. Add the remaining seed and fungicide and stir again. Current recommendations for seed treatment fungicides are given in Table 15.
Crop Rotation
Crop rotation is one of the most effective management tools for control of numerous diseases. Many disease agents survive between wheat crops on wheat residue that was infected the previous growing season. If wheat is planted in the same field the next year, this residue allows the disease agent to infect the next crop. If a crop that is not susceptible to the disease is planted for a period that allows the wheat residue to decompose, the disease agent will die. Crop rotation is most effective for disease organisms that survive in residue and spread only short distances, such as soilborne viruses, leaf and glume blotch and take-all. Rotation is less effective for smuts, powdery mildew and rusts, which are spread over long distances, or the fungi causing Rhizoctonia root rot and Pythium root rot, which survive in soil for long periods.
Disease Resistance
Genetic resistance is the primary means of controlling foliar diseases of wheat (see "Wheat Pest Resistance" chapter). Resistance also does not require additional costs. Very good resistance to powdery mildew and leaf rust has been identified, but these fungi frequently develop new strains. This means that varieties need to be replaced every few years. Resistance to leaf and glume blotch is usually not strong, but varieties with moderate resistance remain so for many years. Moderate resistance to barley yellow dwarf is found in some cultivars. Resistance to soilborne diseases is often not available. However, resistance is available to wheat spindle streak mosaic and soilborne mosaic.
Other Control Practices
Numerous other cultural practices may be effective in controlling specific diseases. Deep tillage may reduce some foliar pathogens, such as Stagonospora nodorum, which survives in residue. However, tillage equipment may also spread certain other diseases such as take-all. Early planting may increase several diseases, because it allows many disease agents to infect plants in the autumn. Early planting favors barley yellow dwarf and take-all. Leaf rust, powdery mildew, and S. nodorum survive through the winter in infected leaves. This allows them to produce spores for new disease cycles early in the spring. Weed control is an important aspect in any management program. It is especially important in the control of volunteer wheat, which may harbor diseases over the summer or allow them to survive in crop rotations not containing wheat. Disease losses can also be minimized by a balanced fertility program that allows the vigorous growth of the wheat plant.
Foliar Fungicides
The use of foliar fungicides and specific recommendations vary depending on the region. Generally, there is no economic benefit from applying fungicides to control powdery mildew and leaf rust when resistant varieties are grown. However, exceptions can occur. Few varieties are highly resistant to leaf and glume blotch and there is some potential for use of fungicides against this disease. Labeled fungicides include mancozeb (Dithane-45 and Manzate 200), benomyl (Benlate) and copper sulfate (Top-Cop, That Big 8), although it seldom performs as well as other materials. Control of leaf and glume blotch with the above fungicides has usually produced yield increases of only 3 to 6 bushels/acre.
Propiconazole (Tilt) and azoxystrobin (Quadris) are labeled for use on wheat and other small grains. They provide excellent control of rusts, powdery mildew, and leaf and glume blotch. Yield increases of 15 to 20 percent on susceptible varieties have been reported. Several restrictions are required, primarily related to the time when grazing can begin. In 1998 propiconazole received approval in Georgia and other states for a 24C label allowing its application up to the fully headed growth stage. Read the label carefully and contact your county agent or refer to an annually updated statewide pesticide handbook for specific recommendations.
Consider using foliar fungicides when disease occurs early in the growing season, favorable weather conditions for disease development are present, and there is a high yield potential. Foliar fungicides should usually not be applied before the late jointing stage. Application before this stage only gives cosmetic control of foliar diseases and reduces effectiveness later in the season when grain is filling and conditions are more favorable for disease.
Table 16. Effectiveness of control methods for wheat diseases*
Wheat disease |
Seed quality
and treatment |
Resistant varieties
|
Crop rotation
|
Foliar-applied fungicides
|
Cultural practices
|
Leaf and glume blotch |
3
|
2
|
1
|
1
|
b, c
|
Leaf rust |
|
1
|
2
|
1
|
a
|
Stem rust |
|
1
|
3
|
3
|
|
Powdery mildew |
|
1
|
2
|
1
|
c
|
Barley yellow dwarf |
|
2
|
2
|
|
a, c
|
Bacterial stripe (black chaff) |
2
|
3
|
2
|
|
c
|
Scab |
2
|
3
|
1
|
3
|
b, c
|
Loose smut |
1
|
2
|
|
|
|
Take-all |
|
|
1
|
|
a, c
|
Pythium root rot |
|
|
|
|
b
|
Wheat spindle streak mosaic and soilborne mosaic |
|
1
|
1
|
|
|
* The absence of a number or letter indicates that this control method is not effective.
1 very effective, 2 moderately effective, 3 slightly effective
a delaying time of planting, b deep tillage, c weed control
INSECT MANAGEMENT AND CONTROL
Wheat is attacked by a number of insects that can cause major losses. The primary insect pests of wheat in the Southeast are aphids and the Hessian fly. Aphids also are very important because they transmit barley yellow dwarf virus. Cereal leaf beetle is a new pest causing increasing levels of damage as it spreads throughout the Southeast. A number of other insects also attack wheat, but these pests occur sporadically. If an insect problem is suspected, proper identification of the pest is crucial. Insect damage can be confused with other types of injury; consequently, scouting and identifying the insect pest are very important.
Pest Biology and Damage
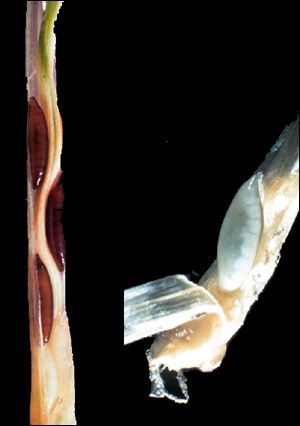
Hessian Fly
The Hessian fly, Mayetiola destructor, was a major factor limiting wheat production throughout the southern United States during the 1980s. In 1989, the Hessian fly destroyed many fields and caused losses of $28 million in Georgia. Widespread use of resistant wheat varieties has greatly reduced damage by this pest. Wheat is the primary host of the Hessian fly, but the insect also will infest barley, triticale, and rye. Hessian fly does not attack oats or ryegrass.
Adults are small black flies about the size of a mosquito. They live about two days during which they mate. Females lay about 200 eggs in the grooves of the upper side of the wheat leaves. Eggs are orange-red, 1/32 in. long and hatch in three to five days. Young reddish larvae move along a leaf groove to the leaf sheath and then move between the leaf sheath and stem where they begin to feed on the stem above the leaf base. Maggots become white after molting and appear greenish white when full grown (Figure 16). Maggots molt into a resting stage (puparia) which is often referred to as the "flaxseed" stage because the pupae resemble seeds of flax. The entire life cycle requires about 35 days at 70°F. Newly hatched larvae are prone to drying while they are exposed on the leaf surface, but once larvae move to the stem base, they are protected from weather extremes.
Maggots suck sap and feeding by a single larva for several days will completely stunt the growth of a vegetative tiller. Stunted vegetative tillers are dark green, do not elongate or produce new leaves, and die after the maggots pupate. Infested jointed stems are short, and the stem is weakened at the joint where feeding occurs. Grain filling of infested stems is reduced, and damaged stems often lodge before harvest. Yield loss usually becomes significant when fall infestations exceed 5 percent to 8 percent infested tillers or spring infestations exceed 20 percent infested stems.
The Hessian fly is a cool season insect and is active during the fall, winter, and spring. The insect oversummers as puparia in wheat stubble. The number of generations during the year is governed largely by temperature. Generally, three to four generations occur in the Piedmont region of the Southeast, and four to five generations occur in the Coastal Plain. Adults emerge from oversummered pupae in wheat stubble about September 1. Because wheat has not yet been planted, the first generation develops entirely in volunteer wheat and weed hosts. Little barley is the only important non-crop host in our area. A second and sometimes a third generation occur in late fall and winter. One generation usually occurs in the spring. The fall and first spring generations stunt and kill seedling plants and vegetative tillers. The spring generation infests jointed stems during stem elongation with larvae feeding between the stem and leaf sheath above each joint (node).
The use of resistant plant varieties has resulted in the development of numerous Hessian fly biotypes. Biotypes are identical to each other and to the parental type, except each biotype contains the ability to overcome a specific set of wheat genes for resistance to the pest. Currently, the H7H8 gene combination is the only effective resistance source in the coastal plain region of the Southeast. New resistance genes are being incorporated and soon will be deployed in new southern wheat varieties.
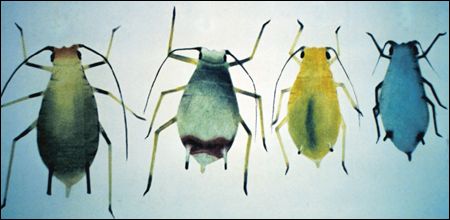
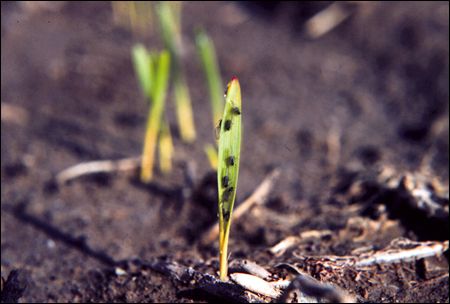
Aphids
Wheat in the Southeast is attacked by a number of species of aphids. These include the greenbug, Schizaphis graminum; English grain aphid, Sitobion avenae; rose grain aphid, Metopolophium dirhodum; yellow sugarcane aphid, Sipha flava, bird cherry-oat aphid, Rhopalosiphum padi; rice-root aphid, Rhopalosiphum rufiabdominalis; and corn leaf aphid, Rhopalosiphum maidis (Figure 17). All of these species attack a wide range of grass hosts including all of the small grain crops. With the control of the Hessian fly by resistant varieties, aphids have become the number one insect pest of wheat in the Southeast.
Aphids cause two types of damage. They directly damage plants by sucking sap and, in the case of the greenbug, by injecting a toxin while feeding. Greenbugs produce yellowed-brownish chlorotic leaf injury at the site of feeding. In general, the greenbug and English grain aphid cause more severe direct damage than the other species. More importantly, aphids can transmit plant disease viruses of which barley yellow dwarf is the most devastating. The bird cherry-oat and other Rhopalosiphum spp. aphids are the most important vectors in our area. Infection during seedling and vegetative stage plants in the fall and winter is much more damaging than infection during the spring. Fall infection stunts plants, increases susceptibility to cold injury, and reduces grain yield of infected plants by about 50 percent (see "Diseases of Wheat" chapter).
Aphids do not have distinct generations, but population numbers are greatest in the fall and spring. Winged adults move from summer grass crops, weeds, and volunteer small grain plants to newly sown fields in the fall (Figure 18).
The winged adult produces wingless forms that feed in clusters on all vegetative parts of the plants and on the grain heads. In the Southeast, aphids overwinter in wheat fields as nymphs and wingless adults. Several aphid species often occur together in the same field. Aphid populations are predominantly greenbug, bird cherry-oat, and rice root aphids in the fall and winter, whereas English grain aphid becomes predominant in the spring.
Aphids are born pregnant; consequently, populations can increase and reach economic levels rapidly. Dry, warm (but not hot) weather promotes rapid population increase. Heavy and violent rainstorms can reduce populations considerably during the spring when aphids are exposed on grain heads. Aphids also are attacked by several species of insect predators and parasites. The most visible predators are several species of ladybird beetles, which can become abundant in the spring.
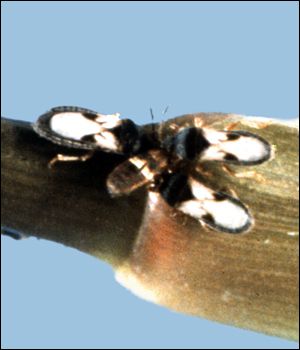
Chinch Bugs
Adult chinch bugs, Blissus leucopterus, are 1/6 to 1/5 in. long and are black with white wings that are marked with a triangular black patch on the outer margins (Figure 19). The white wings give the insect a spotted appearance. Nymphs are brown to reddish with a transverse pale colored band. Both nymph and adult chinch bugs feed on grasses including all the small grain crops by sucking sap. Feeding can discolor and stunt plants, but populations usually are not large enough to cause economic damage on small grains. The insect overwinters as an adult and the entire life cycle takes about 40 days. Chinch bugs avoid damp, shaded areas, therefore, they are usually found along field edges and in thinner stands where sunlight reaches the soil. Additionally, chinch bugs are mainly a problem in dry years. They also may increase in small grain crops in the spring and move, as the wheat matures, to summer annual grass crops such as corn, sorghum, and millet in adjacent fields or to doublecropped plants in the same field. Chinch bugs can be very damaging to double cropped corn, sorghum and millet seedlings, especially under dry conditions.
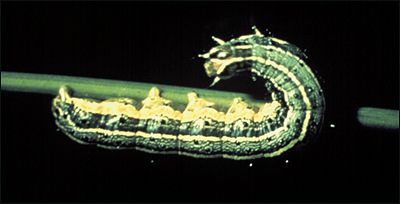
Fall Armyworms
The fall armyworm, Spodoptera frugiperda, beet armyworm, S. exigua, and yellowstriped armyworm, S. ornithogalli, damage wheat in the autumn where they move into wheat as summer crops mature. Fall armyworm infestations usually occur in early planted stands for grazing. Larvae (caterpillars) of these moths are highly variable in size and color (Figure 20). Lengths range from 1/8 to 1½ in. and they are pale-greenish to brown with or without dark stripes along the side and back. Larval size and damage severity vary during the season and between years. Small larvae often produce clear 'window pane' like areas on leaves which normally does not reduce grain yield. Larger larvae mostly eat leaves but can destroy seedling plants. Activity by these armyworms typically is greatly reduced by freezing temperatures, and damage usually is limited to early plantings for forage production.
True Armyworm
The true armyworm, Pseudaletia unipuncta, typically attacks wheat during the stem-elongation and heading stages during the spring. Larvae reside on the soil surface during the day. Larvae climb stalks to chew holes in leaves, eat spike glumes, and sometimes cut seed heads. Damage usually occurs during cool, wet springs, with rank and lodged areas of a field often being most severely damaged. In most years, armyworms are attacked by numerous parasites and several diseases, which prevent them from causing economic damage.
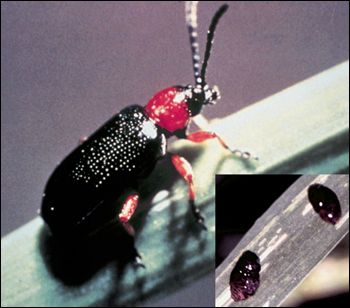
Cereal Leaf Beetle
The cereal leaf beetle, Oulema melanopus, was introduced from Europe into Michigan in the 1950s and is slowly spreading southward. It is now found as far south as central Alabama, Georgia and South Carolina. The immature stage (larva) of cereal leaf beetle feeds on the leaves of wheat, oats and certain other grasses; it prefers oats but also readily accepts winter wheat.
Cereal leaf beetle has one generation per year. Adult beetles spend the winter in the woods and field borders. During the first warm days of spring, the beetles disperse into small grain fields, mate, and begin to lay eggs. Eggs hatch in about seven days, and larvae begin to feed on the cereal leaves. The larvae feed for about three to fourweeks, then leave the plant, and move into the soil. The adults of the new generation come out in late May and early June, feed briefly, then move out of the fields, and remain inactive until the following spring. New generation adults may feed on corn leaves but seldom cause serious damage.
Cereal leaf beetle adults are about 3/16 in. long and 1/16 in. wide (Figure 21). The adults have dark, metallic blue wing covers, orange legs, and an orange collar. The head and the rest of the body are black. Eggs are cylindrical with rounded edges. They are light orange when laid and darken gradually over time to brown. Eggs are usually deposited singly or in rows of two to four on the top side of the leaves. Each female lays from 12 to 50 eggs. Larvae are pale yellow with a brown head and legs. In the field, they look mostly black because they smear excrement over their bodies. People walking through a field infested with cereal leaf beetle larvae may emerge with black stained pants legs because this black coating easily rubs off the larvae. The larvae are about 1/16 in. long just after hatching and 1/3 in. long when fully grown.
Cereal leaf beetle larvae feeding activity results in long, window-like slits in the leaves. Larvae are especially damaging when they feed on the flag leaf. This is because productivity of the flag leaf is closely linked to final yield, however, recent studies indicate wheat can tolerate up to 50 percent flag leaf defoliation before grain yield is reduced.
Check fields weekly from late March through April for defoliation and for adult beetles, eggs, and larvae. Sample the middle of the fields as well as the edges. An insecticide should be applied after most eggs have hatched but before extensive defoliation (>10 percent) has occurred. Cereal leaf beetle has few natural enemies in the southern United States, but exotic parasites of the egg and larval stages are being released throughout the region. Heavy rains can kill larvae, so if heavy rains occur between the time a field is checked and insecticides are to be applied, wait for the foliage to dry and recheck the fields.
Lesser Cornstalk Borer
The lesser cornstalk borer, Elasmopalpus lignosellus, is a moth whose larvae bore into the stem base at or below the soil surface and kills seedling plants in the fall. This insect feeds on many host plants and often moves from weeds and stubble of the previous crop to newly planted small grain plants in the same field. Damage by lesser cornstalk borers usually is restricted to early planted stands for grazing.
Stalk Borers
Several insect species bore into wheat stalks. These species in the Southeast are the wheat stem maggot, Meromyza americana, and European corn borer, Ostrinia nubilalis. Both pests cause grain heads of infested stems to die and bleach white before the rest of the crop matures.
The wheat stem maggot is a small yellow-green fly. The adult is harmless, but the larva, which is green with faint white stripes, tunnels into the stem. The insect usually is present in the fall and overwinters as a full-grown maggot inside a wheat tiller. The wheat stem maggot rarely causes significant damage to wheat in the Southeast.
The European corn borer attacks many crops including corn, sorghum, cotton, and small grains. Adults lay egg masses in wheat in the spring. The larvae are up to 1 in. long and are pale brown with small inconspicuous brown spots. Young larvae feed between the leaf sheath and stem, whereas larger larvae bore into the stem and tunnel in the areas between joints. European corn borers can not be controlled with insecticides once they enter the stem, thus a spray treatment must be applied while the larvae are small. Although the insect produces highly visible damage, at present losses in the Southeast are slight.
Stink Bugs
Large numbers of brown, Euschistus spp., or Southern green, Nezara viridula, stink bugs sometimes infest wheat in the coastal plain region during grain filling to harvest. Stink bugs feed by sucking fluid from developing grain, causing grain to be shriveled. The impact of stink bug feeding injury on wheat has not been determined, but most likely infestations rarely cause economically important damage. Instead, stink bugs disperse from wheat fields at harvest to infest adjacent summer crops where they may cause significant damage.
Thrips
Thrips are very small (¼ in. or less in length) slender-bodied insects either wingless or winged with two pairs of very slender wings fringed with long hairs. Studies in Georgia and Florida found that the predominant species attacking small grains in the Southeast are the tobacco thrips, Frankliniella fusca, and cereal thrips, Limothrips cerealium. Nymphs are variously colored but adults are typically black. Thrips feed between the leaf sheath and stem where they suck plant fluids. Although thrips may become very abundant, they do not cause significant damage in small grains and do not require control in wheat. Wheat is not a host for tomato spotted wilt virus which can be transmitted by tobacco thrips. However, as wheat matures, thrips may disperse to new plantings of adjacent summer crops where they can cause direct feeding damage.
Management Tactics
Plant Resistance
The most efficient and economical method for controlling the Hessian fly is the use of resistant varieties. Many varieties currently available are resistant in the Coastal Plain region. Wheats grown in the Southeast do not contain high levels of resistance to any other insect pest.
Cultural Practices
Several cultural practices can aid in the management of insect pests in wheat. Most insect pests, including the Hessian fly, aphids, fall armyworms, and others can become established in a field on volunteer wheat growing in the summer annual crop before wheat planting. Therefore, control of volunteer wheat by reducing combine losses of grain at wheat harvest and effective subsequent weed control will help in reducing early pest buildup on volunteer wheat. Tillage can have a large impact on fall populations of insects in wheat. Insect populations and damage generally are greater under no tillage than under conventional tillage systems. Table 17 shows the effect of moldboard plowing on fall Hessian fly infestations in wheat. Fall infestations were almost three times greater in the no-till than the plow tillage systems. Plowing buries wheat stubble where Hessian flies oversummer and suppresses volunteer wheat in the late summer. No tillage also may enhance fall populations of aphids, which are attracted to the plant stubble.
Table 17. Effect of moldboard plowing on Hessian fly infestation in the fall and spring
Tillage Treatment | % Infested Tillers | |
Fall | Spring | |
Plowing (fall and spring) | 8 | 40 |
Plowing (fall only) | 7 | 44 |
No-tillage | 23 | 43 |
Generally, insect damage is more severe in early wheat plantings. Early plantings allow insects to become established and increase before freezing temperatures limit activity. Damage by many insects can be minimized or avoided by not planting before the recommended planting date in your area.
The effect of planting date on Hessian fly populations in wheat is shown in Table 18. Fall infestations decline in later planting dates. Therefore, damage by the Hessian fly may be minimized by timely planting, but fall damage probably will not be eliminated, particularly in the coastal plain region where activity can occur throughout the winter.
Table 18. Effect of planting date on Hessian fly infestation in winter wheat at Plains, GA
Planting Date |
% Infested Tillers
|
||
Dec. 5
|
Feb. 9
|
May 12
|
|
Oct. 23 |
42
|
24
|
65
|
Nov. 5 |
16
|
23
|
70
|
Nov. 20 |
0
|
20
|
77
|
Dec. 5 |
—
|
2
|
70
|
Early planting also enhances fall aphid infestations and infection of barley yellow dwarf virus. Wheat planted early for grazing by livestock is particularly vulnerable to attack by insects, especially the Hessian fly, aphids, fall armyworm, lesser cornstalk borer, and grasshoppers. Either rye, oats or a Hessian fly-resistant wheat should be planted for grazing.
Burning stubble in the spring and fall grazing may reduce insect populations somewhat, but these practices are not very effective in controlling most insects including the Hessian fly. Rotation of wheat with other crops also suppresses insect damage somewhat, but insects are highly mobile; consequently crop rotation may not be as effective as it is for less mobile wheat pests.
Biological Control
All wheat pests are attacked and regulated to some extent by a complex of natural enemies. Several non-stinging, parasitic wasps attack and kill Hessian fly larvae. Platygaster hiemalis attacks Hessian fly larvae in the fall and winter, and several other parasitic wasps attack the spring generation. Because of the number of generations, parasites cannot control the Hessian fly during an outbreak year, but natural enemies probably provide long term regulation of Hessian fly populations.
Aphids also are attacked and killed by parasitic wasps, which cause aphids to become light brown "mummies." Several species of ladybird beetle adults and larvae are important predators of aphids. Ladybird beetle adults move into wheat fields from overwintering sites usually in March or early April where they feed voraciously on aphids and often control aphid infestations. This is too late to prevent transmission of barley yellow dwarf virus but may prevent direct aphid injury to developing grain. Hover fly larvae also can be found eating aphids in wheat fields.
Cereal leaf beetles are controlled by parasitic wasps in the northern United States. In 1995, the USDA Animal and Plant Health Inspection Service began releasing a stingless wasp, Anaphes flavipes, that attacks cereal leaf beetle eggs. Nursery plots also have been established to release larval parasites, primarily Tetrastichus julius. Hopefully, once established, these parasites will keep cereal leaf beetle populations below economically damaging levels. Ladybird beetles are also important predators and can destroy many eggs and larvae.
True armyworms and most other wheat pest are attacked by a complex of parasites, predators, and pathogens that prevent their populations from reaching economic levels in most years. Pathogenic fungi are especially important in suppressing populations of chinch bugs, grasshoppers and aphids. These fungi require wet, humid conditions to develop, consequently, populations of these pests typically are worse in dry than wet years.
Insecticides
Insecticide use in small grains is increasing as wheat is grown more intensively. The granular insecticides DiSyston and Thimet (phorate) applied at 1.0 lb active ingredient/acre at planting are effective in controlling Hessian flies for about 45 days on susceptible varieties. These insecticides, however, must be applied in-furrow at planting. Broadcast applications are not effective. Although insecticides applied at planting may control initial Hessian fly damage, these treatments will not prevent reinfestation by subsequent generations during the winter and spring. Foliar applications of insecticides in the spring for Hessian fly control are highly variable in effectiveness and are not recommended.
The incidence of barley yellow dwarf also may be reduced by controlling aphids in the fall and late winter using foliar insecticides or by using an insecticide seed treatment at planting. The cost of these treatments should be weighed against the historic or expected loss from aphid infestation and barley yellow dwarf infection. Except for the Hessian fly and lesser cornstalk borer, all other insect pests including aphids can be controlled by applying foliar insecticides when population numbers exceed economic thresholds (Table 19). Consult the Georgia Pest Management Handbook, the Alabama Small Grain Insect, Disease and Weed Control Recommendations, or your local county Extension office for specific insecticide recommendations.
Table 19. Damage symptoms and economic thresholds of insect pests of wheat
Insect |
Damage Symptoms | Treatment Threshold1 |
Season Long | ||
Aphids | Suck plant sap and may cause yellowing and death of leaves; reduce grain size when grain heads infested; transmit barley yellow dwarf virus. | Seedlings: 2-3/row ft, 30-60 days; after planting: 6/row ft; 6-10 in. plants: 1 to 2/tiller; boot to heading: 5/stem; heading to dough stage: 10/stem; hard dough to maturity: damage not economic. |
Hessian fly | Vegetative plants: stunted tillers dark green, tiller death. Jointed stems: stunted, weakening of stem at point of feeding injury, reduced grain size and weight. Infested stems may lodge before harvest. | Use resistant varieties; on susceptible varieties use granular insecticide at planting. |
Chinch bugs | Suck plant sap causing discoloration. | Seedlings: 1-2 adults/5 plants; Spring: 1 adult/stem. |
Autumn - Seedling Stands | ||
Fall armyworm, beet armyworm & yellowstriped armyworm | Small larvae cause “window pane” feeding on leaves which is not economically important; larger larvae consume leaves and entire plants; damage usually in early plantings. | Three or more larvae/square ft |
Grasshoppers | Destroy leaves of seedlings during fall. Damage usually along field margins. | 3-5/square yd within the field |
Flea beetles | Pin hole defoliation of seedlings in fall. Damage common along field margins. | Seedling defoliation not economic unless complete defoliation occurs. |
Lesser corn stalk borer | Larvae bore into base of seedlings in fall. Usually only in early plantings. | Not established. |
Spring - Stem Elongation and Grain Fill | ||
Cereal leaf beetle | Adults and larvae chew long, narrow holes in leaves; larvae usually leave lower leaf surface intact; defoliation in spring during heading and grain development. | 0.5 larvae or adult/stem. Treated after most eggs have hatched except with Warrior/Karate, which can be applied at 50 percent egg hatch. |
Mites | Suck plant sap; cause leaf discoloration. | Treat when leaf discoloration appears over large areas of a field. |
Thrips | Suck plant sap; may cause slight discoloration of leaves; may disperse to adjacent summer crops. | Injury not economic; do not treat. |
Stink bugs | Bugs suck fluids from developing grain; infest grain at harvest; may disperse to adjacent summer crops. | Not established. |
European corn borer | Small larvae chew holes in leaves; large larvae tunnel in stem killing developing grain head which become bleached white. | 5 percent infested stalks; treat when larvae are small and numerous; larvae can not be controlled once they bore into a stem. |
Wheat stem maggot | Maggots tunnel into stem in the fall. | Not established. |
Summary of Management Practices
- Avoid continuous planting of wheat in the same field.
- Select a Hessian fly resistant variety.
- Control volunteer wheat.
- If possible, chisel plow and disk harrow fields to bury wheat debris.
- Do not plant wheat for grain before the recommended planting date for your area.
- Consider planting rye, oats, or ryegrass instead of wheat for grazing.
- Granular insecticides applied in-furrow at planting should be considered for Hessian fly susceptible varieties. Do not apply DiSyston 15G to wheat grown for grazing.
- Scout fields (sample 5 to 10 sites per field) for insect pests, except Hessian fly, and control with foliar applied insecticides when numbers exceed treatment thresholds (Table 19).
WHEAT PEST RESISTANCE
Varietal selection should be based on many factors including resistance to insects and diseases. Breeders traditionally add the best combinations of pest resistances to the highest yielding parents, providing each new variety with the most desirable characteristics available. When appropriate and possible, combine pest resistance with other control practices, such as crop rotation, because they tend to complement and enhance one another. Use of resistant varieties is preferable from both economic and environmental viewpoints and has a sound history of successes.
Types of Resistance
Disease Escape
Although not a true type of genetic resistance, certain characteristics of a variety can reduce or prevent pest damage. Some varieties mature early enough to avoid damage from pests. This escape mechanism is effective during many seasons with diseases such as stem rust caused by Puccinia graminis. Dry spring weather or the absence of infection-promoting dews slows the progress of leaf and glume blotch caused by Stagonospora nodorum and leaf rust caused by Puccinia recondita. Early maturing varieties often have a better chance of escaping losses. Planting late in the recommended period when temperatures are cooler reduces the likelihood that aphids will transmit barley yellow dwarf virus to seedlings and generally avoids seedling damage by insects.
Genetic resistance to pests may occur in several different forms:
Hypersensitivity
Many plants respond to infection with a biochemical reaction that kills the cells surrounding the infected area and inhibits the further development of the causal microorganism. This hypersensitive resistance has been used extensively for resistance to rusts and powdery mildew (Blumeria graminis). A related phenomenon characterizes plant resistance to the Hessian fly (Mayetiola destructor). Fly larvae that feed on resistant wheat plants are killed by a chemical in the plant before they can cause any damage.
Tolerance
Tolerant plants have the capacity to yield well in spite of infection by disease or infestation by insects. This tolerance protects the plant from the damaging effects of the pests even though it is severely infected. Protection derived from this mechanism is difficult to identify. Barley yellow dwarf is a disease to which some varieties exhibit tolerance. However, plant tolerance can be overwhelmed by severe disease infection or very large pest numbers.
Partial Resistance
Several to many genes control a complex sequence of responses to infection in some plants, which serve to slow the development of a disease such as leaf and glume blotch. This resistance may also restrict the size of the area infected, lengthen the reproductive cycle of disease-causing micro-organisms, and greatly reduce the number of fungal spores produced. Partial resistance slows disease development enough to allow the plant to mature without suffering significant yield loss. This slow resistance often lasts longer than the hypersensitive type, thus prolonging the useful life of a variety.
Resistance to Specific Pests
Leaf Rust
Leaf rust populations comprise several different strains or races, which differ in their capacities to survive on various wheat varieties. Thus, when a new rust resistant variety is introduced, it provides protection from losses only until the rust population changes. Then a new race often builds up that overcomes the plant's resistance and causes significant damage. This limits the useful life of a new variety to as little as two to four years in the Southeast. Breeding programs must maintain a continuous effort to incorporate new sources of resistance to leaf rust. Slow rusting wheat types (partial resistance) have been discovered which may also last longer than the conventional types with one or two effective genes. These are genetically complex and hence difficult to incorporate quickly into new varieties. Generally, the newest varieties should provide the best levels of resistance to leaf rust. Data from comprehensive regional surveys of the rust population guide selection and use of new sources of resistance.
Powdery Mildew
Powdery mildew, like the rusts, is caused by a fungus that readily evolves new races that can quickly render resistance ineffective. Several single genes have been identified and used in the Southeast to provide protection, but most have eventually been overcome by new races. Yield losses of 34 percent have been documented. Partial resistance, sometimes called "slow mildewing," may be an important type of resistance that would not be outdated by the occurrence of new races. New varieties feature the most current and effective genes for resistance to powdery mildew.
Leaf and Glume Blotch
Resistance to leaf and glume blotch is the partial type which slows the infection process, delays the time until lesions and new fruiting bodies form, and reduces the amount of spores produced. Varieties such as Gore with this type of resistance slow the development of the epidemic and protect the plant from damage. The level of resistance remains virtually constant over time.
Hessian Fly
Genetic resistance to Hessian fly is available and can effectively eliminate economic losses. Unfortunately, this type of resistance is often rendered ineffective by new biotypes of the insect, which are similar to races of plant disease agents. Resistance genes offer differing levels of protection based upon the predominant biotypes in the area. Gene pair H7H8 currently offers good protection in the Southeast. New genes are being incorporated into varieties as shifts in the Hessian fly occur. Most varieties now grown in the Southeast are resistant.
Barley Yellow Dwarf
Resistance to barley yellow dwarf virus is often difficult to determine, especially in wheat, because symptoms are variable, and sometimes infected plants may exhibit no or only mild symptoms. Some wheat varieties exhibit a degree of tolerance to barley yellow dwarf. Yield loss is not always associated with the severity of symptoms. The best method to determine tolerance to barley yellow dwarf virus is to compare the grain yield of infected plants of a variety with those protected with insecticides to prevent or reduce transmission of the virus by aphids.
CROPPING SYSTEMS FOR PEST MANAGEMENT IN SMALL GRAINS
Crop rotation has long been a valuable management practice to control weeds, insects and diseases. Many plant pathogenic fungi and bacteria survive on infected debris of a crop. When the plant tissue decays, the pathogens also die. Crop rotation often results in the loss of the preferred host or the only host for an insect or plant pathogen. Pest populations then decline below threshold levels needed to cause economic damage. Weeds may be less able to compete for nutrients or to thrive in low light conditions under a dense crop canopy of rotational crops. In most cases, the longer the period between planting of the same crop, the better the pest control. Crop rotation also can have beneficial effects on soil tilth, soil organic matter and plant nutrition. The best example of a nutrient benefit is the fixation of nitrogen when legumes are grown and the soil nitrate levels available to a following crop increase. Unfortunately, rotation with a different crop each planting cycle is not always feasible. Much of the small grain acreage in the Southeast is grown as part of a doublecropping system. Therefore, rotations must be planned for two crops a year in many cases. In the past, an option was to leave fields fallow to manage pests and rebuild soil structure. The economic pressures of modern agriculture make this less of an option. The optimum is to grow a diversity of crops with a positive economic benefit derived from each crop in the rotation.
Below is a summary of recommendations based on current research for management of small grain pests with crop rotation and soil management. All crops in a rotation benefit from reductions in pest populations.
A three year study of crop rotation effects on pests using the wheat:soybean doublecropping system was conducted at the Southwest Branch Station at Plains, GA. Twelve rotation sequences were planted. Canola was substituted in some rotations for wheat and grain pearl millet was planted in place of soybean in some rotations. One of the diseases studied was take-all root rot of wheat (see "Diseases of Wheat" chapter). Take-all often becomes severe when wheat is planted more than two consecutive years. Crop rotation is the primary means to control this disease. One year of canola between wheat crops reduced take-all severity to a low level, and grain yield was the same as wheat with no take-all. Soybean as a summer crop does not reduce take-all, but sorghum reduces the disease in a following crop of wheat. Take-all damage after pearl millet was the same as after soybean. Rye and barley are less susceptible to take-all than wheat, but wheat sustains as much damage after these crops as after continuous wheat. Oats is the only small grain that can be rotated with wheat to reduce take-all. Wheat should not be grown for more than two consecutive years. Both canola and pearl millet are compatible with the wheat:soybean system. Grain pearl millet has potential for the expanding poultry industry in the Coastal Plain. The most critical time in the cropping system is the planting time for canola, which with current cultivars, must be completed by November 10 in the Coastal Plain. Therefore, choice of a soybean cultivar in the proper maturity group is important. Pearl millet matures in September, so planting of the autumn crop is more flexible. Continuous wheat also favors soilborne wheat mosaic and wheat spindle streak mosaic (see "Diseases of Wheat" chapter).
Hessian fly is the major insect pest of wheat. The primary means of control is plant resistance, but crop rotation also is useful if susceptible cultivars are planted. Other small grains are moderately susceptible and can serve as a source of Hessian fly for wheat. Hessian fly declined when canola was rotated with wheat.
With the increase in cotton acreage, tests have been conducted to determine if planting cotton after wheat is possible. In Florida, early to medium-maturing cotton cultivars planted after wheat have yielded 1,000 lb lint/acre. Availability of irrigation and tillage need to be considered as part of cotton management after wheat. Cropping systems using rye or other small grains as a winter cover crop or for winter grazing followed by full-season corn, soybeans or cotton are options. Small grain winter cover crops can reduce nematode populations which affect summer crops. No-till cotton planted into rye residue produced three bales of cotton per acre in large plots in Piedmont soils at Watkinsville, GA. Intercropping cotton into wheat prior to wheat harvest has been tested in South Carolina. With proper equipment and row spacing, cotton was successfully planted into wheat. The expansion of vegetable acreage also provides a system that may include rotations with wheat or other small grains.
Fusarium head scab of wheat causes direct losses in yield and seed quality but is most important because of toxins that affect humans and animals produced by the fungus. The same fungus causes a stalk rot of corn. Scab has become a serious problem in the upper Midwest in recent years because of higher levels of precipitation and use of reduced tillage following corn. Wheat scab has not been as serious in the Southeast. However, the potential for scab is present whenever wheat follows corn or possibly sorghum. Corn and sorghum residues should be thoroughly incorporated into soil prior to planting wheat.
If wheat or other small grains are planted in consecutive years, deep tillage before planting reduces surface residue that harbors Hessian fly pupae and pathogens such as Stagonospora nodorum. Deep tillage also incorporates grassy weeds that harbor aphids that damage seedlings directly or transmit barley yellow dwarf virus.
HARVESTING, DRYING AND STORAGE
Wheat must be harvested with minimum losses to realize the maximum return from all production practices. Minimum losses are achieved by harvesting on time with proper combine adjustments and operation. If the grain contains excess moisture, it must also be dried for safe storage.
Combine Functions
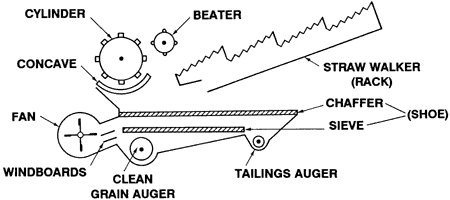
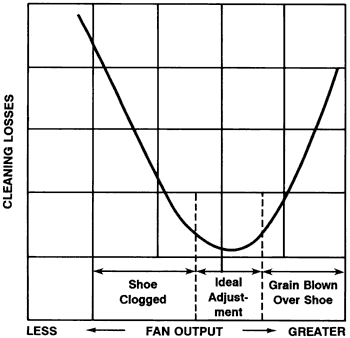
A thorough understanding of the combine components is required to make the proper adjustments for satisfactory operation (Figure 22). By studying this diagram, you should be able to understand the general functions of the combine and why adjustments must be made for various conditions.
The header gathers, cuts and conveys the grain and straw into the combine, then into the cylinder. All the grain and straw must then pass between the cylinder and concave, where the grain is threshed from the heads. Some of the threshed grain will fall through the concave onto the cleaning shoe, which is made up of a chaffer (coarse sieve) and a sieve (fine sieve).
The straw and the grain that remains in the straw then move to the straw walker (rack). The reciprocating motion of the straw walker causes the straw to move across the straw walker and out the back of the combine. As the straw moves over the straw walker, the threshed grain is vibrated out of the straw and conveyed to the chaffer where it joins the threshed grain coming directly from the cylinder and concave.
The grain contains a lot of trash and broken straw when it reaches the chaffer. The chaffer is a coarse sieve that is usually adjustable. The chaffer shakes in a back-and-forth motion, which moves the material riding on the chaffer out the back of the combine. Grain and heavy small trash fall through the chaffer onto a sieve that is finer than the chaffer. The sieve also shakes, causing the material on the sieve to move to the rear. The material passing over the sieve is called "tailings" and is returned by an auger and elevator to the cylinder for additional threshing.
A fan blows air up through the sieve and chaffer to remove light trash and to aid the rearward movement of the material on the chaffer and sieve. Excess cleaning air will blow grain out the rear of the combine, and too little air may cause the cleaning chaffer to clog and pass grain out the back of the combine (Figure 23).
Another method of threshing is to place one or two rotors (rotating cylinders) parallel or transverse to the flow of the crop through the threshing area. Threshing takes place as the material moves towards the rear in a spiraling cylindrical mass between the rotor and the rotor cage.
The crop makes several revolutions around the chamber while passing through the machine but at a slower speed than the rotor. As a result, the rasp bars make several contacts as the crop is moved past the concaves. Thus, the rotor uses a rubbing, rolling action in threshing. Separation of the grain from the straw is aided by the centrifugal force set up by the rotors. Nearly 85 percent of the threshed grain falls through the concave grate. The remainder of the grain, along with the straw, continues the rearward spiraling motion into the separation area of the rotor cage.
Combine Adjustments
It is evident that one adjustment can affect grain losses in several ways. The main combine adjustments are the reel position and speed, the cutter bar height, the cylinder speed, the concave clearance, the chaffer and sieve openings, and the fan and windboard settings. The owner's manual will give the initial settings for all grain; however, the following adjustments are for small grain in average conditions.
The final adjustments must be made in the field because they will be affected by such things as grain moisture, the condition of the straw, the quantity of weeds or green material, the kind of grain and yield.
Reel
The reel shaft should be 8 to 12 in. forward of the cutter bar with the top of the reel slats (boards) at a height just below the grain head. The reel tip speed should be 25 percent faster than the ground speed. For lodged wheat, a pickup reel should be used; its height should just clear the cutter bar.
Cylinder Speed
Tip speed of 5,700 to 6,500 feet per minute is recommended for wheat. The cylinder revolutions per minute (rpm) will vary with its diameter as follows:
12 x tip speed (ft/min) 3.14 x diameter (in) |
= | 3.82 x tip speed (ft/min) Cylinder diameter (in) |
The cylinder speeds required for an initial cylinder tip speed of 6,000 feet per minute with various cylinder diameters used in new combines are shown in Table 20.
Table 20. Suggested initial cylinder speeds
Cylinder diameter (in) | Cylinder speed (rpm) |
18.0 | 1,273 |
19.3 | 1,187 |
21.7 | 1,056 |
22.0 | 1,042 |
23.6 | 917 |
![]() |
![]() |
![]() |
Concave Clearance
Concave clearance should be ¼ to ½ in. with an initial setting of 3/8 being suggested (Figure 24). Consult your owner's manual for rotor speeds in rotary combines.
Chaffer
Set chaffer opening to 5/8 in. (adjustable type) measured perpendicular to the tip of the movable finger (Figure 25).
Sieve
Set sieve opening at ¼-in. (adjustable type) measured perpendicular to the tip of the movable finger (Figure 25).
Fan
Inlet 75 percent open with windboard directing air to the front half of the chaffer and sieve. Note: On models with an adjustable speed fan, start with an initial fan speed of approximately 65 percent full speed.
Ground Speed
Three miles per hour maximum.
Combine Losses
The grain tank should be checked periodically, along with the combine losses on the ground, to make sure the combine is adjusted properly. Excess trash in the grain tank means improper fan, chaffer and/or sieve adjustment. If you try to get the grain too clean, you will increase losses by blowing grain out the back of the combine. Also, check the tailings elevator for the quantity of grain being returned to the cylinder. Excess tailings can result in cracked grain.
Field losses may be divided into two categories: preharvest and harvest (machine). Preharvest losses are defined as those shatter losses on the ground and the loss of dry matter due to birds, wildlife, weather, and natural respiration. Figure 26 illustrates the effect of time on these preharvest losses.
Harvesting losses are those losses caused by the combine. Some harvesting losses are unavoidable, but excessive losses are due to improper combine operations and/or adjustments. Modern combines are capable of harvesting 97 percent to 98 percent of the small grain.
The harvesting machine losses can be divided into the following categories:
Header Losses
The grain lost by rough handling by the cutter bar and the reel, and grain not cut by the cutter bar because of excessive cutting height or lodged grain.
Cylinder Losses (threshing loss)
The grain lost over the straw walker because of unthreshed heads and/or cracked grain from high cylinder speeds and/or insufficient concave clearance.
Combine Leakage Losses
Grain lost through cracks and clean-out doors in the bottom of the combine.
Straw Walker Losses (rack or separating losses)
Threshed grain lost over the straw walker because of excessive straw feed rate caused by excessive ground speed and/or low cutter bar height.
Chaffer and Sieve Losses (shoe or cleaning losses)
Grain lost over the chaffer and sieve because of excess fan air (grain blown over), excess material on the chaffer (straw broken up too much by the cylinder because of excessive speed and/or too little concave clearance), or because the chaffer and sieve are closed too much (material riding over the chaffer out the rear of the combine and excessive amount of tailings returned to the cylinder).
Checking Losses
Field losses can be determined by counting the kernels of grain on the ground for a given area (Table 21). The number of kernels per square foot should be counted in several places and averaged for more accuracy. There are usually more kernels directly behind the combine than across the rest of the swath; therefore, when determining threshing losses, the kernels counted behind the combine must be taken across the entire swath width (Figure 27).
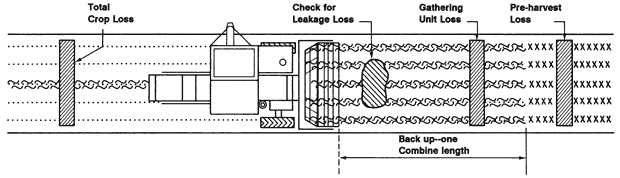
Table 21. Small grain loss chart
Crop |
Kernels per sq ft per bu per acre
|
Barley |
14-15
|
Oats |
10-14
|
Rye |
22-23
|
Triticale |
11-13
|
Wheat |
17-20
|
Combine losses can be checked by the following procedure:
- Disengage the straw spreader if one is being used.
- Harvest a 300-foot-long strip where the grain is typical of the entire field.
- Stop the combine and let it clean out.
- Mark the position at the rear of the header and just in front of the combine rear wheels.
- Back the combine one length and check for losses as described below and indicated in Figure 27.
- Check for any leakage loss at the previously marked position in front of the rear wheels. A heavy streak of grain indicates a grain leakage. Repair the leakage hole in the combine and restart the loss testing at Step 2.
- Count the shattered kernels on the ground in a 10 square foot area across the width of the header in front of the combine in the uncut grain area. Enter the total number of grains counted in Table 22, Column 1, Line A. This represents the preharvest loss.
- Count the total number of kernels on the ground including the kernels in any uncut heads in a 10 square foot area across the width of the header at the previously marked position at the rear of the header. Enter the total number of kernels counted in Table 22, Column 1, Line B. This represents the header losses plus the shatter losses.
- Count the total number of kernels on the ground including the kernels in any uncut or unthreshed heads in a 10 square foot area across width of the header in its backed position. Keep a separate count of the kernels in any cut but unthreshed heads (cylinder loss) and enter the number of kernels found in the heads in Table 22, Column 1, Line C. Be sure also to include these in the total count, and enter the total number of kernels in Column 1, Line D.
- Complete Table 22 to determine the losses. Complete each line in Column 3 by dividing the number of kernels found in Column 1 by the number of square feet from Column 2. Complete Lines A, B, C, and E in Column 4 by entering the kernel count for the specific small grain from Table 21. Complete Lines A, B, and C, in Column 5 by dividing the average number of kernels from Column 3 by the number of kernels per square foot equivalent to 1 bushel per acre from Column 4. Complete Lines E, F, G, H, I and J, as indicated in the table.
The cylinder loss (cut but unthreshed heads, Table 22, Column 5, Line C) should not exceed 0.5 percent of the yield, and the total machine loss should not exceed 3 percent of the yield. If the total machine losses are over 3 percent, the appropriate adjustments should be made and the losses remeasured.
Table 22. Grain loss data
Line | Location and type of loss |
Col. 1
|
Col. 2
|
Col. 3
|
Col. 4
|
Col. 5
|
Total kernels found
|
Total area checked* (sq ft)
|
Average number of kernels per sq. ft. (Col. 1 ÷ Col. 2)
|
Average number of kernels per sq. ft. per bu per acre (Table 20)
|
Loss
(bu per acre) (Col. 3 ÷ Col. 4) |
||
A | Uncut grain (shatter loss) |
10
|
|
|
|
|
B | Underheader (header plus shatter loss) |
|
10
|
|
|
|
C | Cut unthreshed kernels (cylinder loss) |
|
10
|
|
|
|
D | Behind the combine (unadjusted total loss) |
|
10
|
|
|
XXXXXX
|
E | Unadjusted separation and cleaning loss |
(Col. 3, Line D - Col. 3, Line B) = (Col. 4, Line D)
|
||||
F | Header loss |
(Col. 5, Line B) - (Col. 5, Line A)
|
||||
G | Cylinder loss (threshing loss) |
(Col. 5, Line C)
|
||||
H | Separating and cleaning loss |
(Col. 5, Line E)
|
||||
I | Total machine loss |
(Line F) + (Line G) + (Line H)
|
||||
J | Total loss |
(Col. 5, Line A) + (Line I)
|
Drying and Storage
Wet grain in grain bins must be dried to be stored. Small grain normally contains a relatively small amount of excess moisture. Bin drying using the layer-in-bin method is generally the most economical. A volume greater than 25,000 bushels is generally required before a batch or continuous flow dryer is indicated.
Equipment for Layer-in-bin Drying
Adequate drying equipment is necessary to satisfactorily dry grain. With the larger harvesting machines commonly available, drying speed is the main problem with in-storage or layer, drying. Speed is achieved by using either larger quantities of air, or more heat, or both. Heat will overdry the grain, so large quantities of air are necessary for selecting equipment for satisfactory and economical operations.
Table 23. Typical drying equipment data for grain bins (all specifications from one manufacturer)
Bin diameter (ft)
|
Capacity* (bu)
|
Minimum fan air flow (cfm)
|
Approx. fan power (hp)
|
Heater rating (Btu)
|
Roof Exhaust
|
||
Entrance area
(sq. ft.) |
Number of manholes
|
Vent open area (sq.ft.)
|
|||||
14
|
1,970
|
3,846 @ 2 in
|
2
|
65,000
|
2
|
1
|
4
|
18
|
3,250
|
6,358 @ 2 in
|
3½
|
105,000
|
3
|
2
|
6
|
21
|
4,450
|
8,654 @ 2 in
|
5
|
140,000
|
4
|
3
|
9
|
24
|
5,800
|
11,304 @ 2 in
|
7½
|
185,000
|
5
|
3
|
11
|
27
|
7,350
|
14,306 @ 2 in
|
12
|
235,000
|
7
|
4
|
14
|
30
|
9,050
|
17,662 @ 2 in
|
15
|
290,000
|
9
|
5
|
18
|
36
|
13,025
|
25,430 @ 2 in
|
20
|
400,000
|
12
|
7
|
25
|
* 16-foot depth from drying floor to eaves.
Fan
The fan should provide at least 25 cubic feet per minute (cfm) per square foot of bin floor area at a static pressure of 2 in. (water column). Typical total quantity of air for various bin sizes is shown in Table 23.
Heater
The heater should be capable of heating the air delivered by the fan 15°F with the bin full or with the fan operating against 3 in. static pressure. (Caution: As the bin is filled, the fan forces less air through the greater depths and less heat is needed.) The heater rating to give a 15° heat rise is shown in Table 23.
Roof Exhaust
The bin should have 1 square foot of open exhaust area for each 1,000 cfm, or one manhole for each 3,000 to 4,000-cfm fan capacity. Because one manhole is standard equipment on a bin, extra manholes must be purchased with all drying bins 18 feet or more in diameter. Inadequate air exhaust area will restrict the fan and reduce air flow (drying capacity). See Table 23 for the exhaust area needed for various bin sizes.
Air Entrance
Entrance collars, the fan-to-bin transition duct, and the drying floor supports adjacent to the fan should not excessively restrict the air entrance to less than 1 square foot open area for each 1,500 to 2,000 cfm. Do not place concrete blocks in front of the air entrance. Use special steel floor supports in front of the fan entrance. If the overall drying floor is supported with concrete blocks, only heavy-weight concrete blocks that have been fully cured should be used.
Table 23 summarizes typical drying equipment specifications. It is much easier and cheaper to purchase equipment meeting these specifications than to find that the equipment is unsatisfactory or does not perform as desired.
Drying For Immediate Sale or Storage
If the crop is being dried for immediate sale or for short-term storage (30 to 60 days), the moisture content should be reduced only to the extent necessary to meet market grade.
Because grain standards no longer relate moisture and grade, determine what is the maximum moisture acceptable without dockage. For example, if you determine wheat can be sold without dockage at 13.5 percent, then dry only to that level. Any further drying is money lost. If the grain is to be stored for very long, it must be dried further. Maximum grain moisture contents considered safe for 12-month storage for all small grains are 12 percent in northern Georgia and 11 percent in South Georgia. Moisture contents lower than these would, of course, be safer. The maximum storage moisture contents require management and aeration during storage. Reduce by 1 percent for poor quality grain, such as grain damaged by blight, drought, etc. Reduce by 2 percent for non-aerated storage.
Air Required for Drying
Because very little heat is used for in-storage drying, the excess moisture is removed mainly with natural air. The air flow must be sufficient to remove the excess moisture before the grain molds or deteriorates. Higher moisture content in the grain requires more air flow. The minimum air flows for various moisture levels are shown in Table 24.
Table 24. Minimum air flow rate for drying (cfm/bu)
Crop |
20% moisture
|
18% moisture
|
16% moisture
|
14% moisture
|
Wheat |
4
|
3
|
2
|
1.5
|
Barley |
4
|
3
|
2
|
1.5
|
Oats |
2.5
|
2.2
|
1.5
|
1.0
|
Rye |
4
|
3
|
2
|
1.5
|
Triticale |
4
|
3
|
2
|
1.5
|
Air Flow Through Grain
Increasing the depth of grain in a bin allows less air flow because of the higher static pressure from the added grains. Less air flow means longer time required for the drying front to move through the wet grain. Larger, more powerful fans provide more air than smaller, lower horsepower fans; but once the fan is purchased and installed the air flow rate per bushel of wet grain is regulated or maintained by varying the depth of wet grain in the bin in relation to the total grain depth. Minimum air flow rates per bushel and maximum grain depth recommendations for in-storage drying are shown in Table 25. A grain bin equipped with a drying fan of the recommended size and sufficient roof exhaust (manholes) will give the minimum air flow for any moisture condition at the maximum first-layer depth shown in Table 26. When this layer becomes dry, another layer two-thirds as deep as the previous layer can be added on top of this first layer. As each layer becomes dry, another layer is added; but the depth of each succeeding layer should be reduced by approximately one-third to maintain the same air flow per bushel of wet grain.
Table 25. Minimum air flow rates and maximum first-layer depths for in-storage drying (Drying depths below are for the recommended fan sizes shown in Table 22.)
Moisture content (%)
|
Air flow required (cfm/bu)
|
Maximum depth
of first layer (ft)* |
Approx. static pressure (in)**
|
Wheat
|
|||
20
|
4
|
3
|
1.4
|
18
|
3
|
4
|
1.5
|
16
|
2
|
5
|
1.9
|
14
|
1.5
|
6
|
2.0
|
Barley
|
|||
20
|
4
|
5
|
1.4
|
18
|
3
|
6
|
1.6
|
16
|
2
|
7
|
1.3
|
14
|
1.5
|
8
|
1.3
|
Oats
|
|||
20
|
2.5
|
6
|
1.9
|
18
|
2.2
|
7
|
2.3
|
16
|
1.5
|
8
|
1.7
|
14
|
1.0
|
10
|
1.8
|
*Depths must be reduced if grain contains fines.
**Pressures given include 0.25 in. for duct losses.
Table 26. Equilibrium moisture contents of grain*
Relative Humidity (%)
|
% Grain moisture content at 77°F
|
|||
Barley
|
Oats
|
Rye
|
Wheat
|
|
15
|
6.0
|
5.7
|
7.0
|
6.3
|
30
|
8.4
|
8.0
|
8.7
|
8.6
|
45
|
10.0
|
9.6
|
10.5
|
10.6
|
60
|
12.1
|
11.8
|
12.2
|
11.9
|
75
|
14.4
|
13.8
|
14.8
|
14.6
|
90
|
19.5
|
18.5
|
20.6
|
19.7
|
*Data not available for triticale
The rule of reducing the depth of each succeeding layer by one-third assumes that the maximum depth is added each time and that the initial moisture content remains constant. If less than the maximum depth is used or if the grain for the upper layer has a sufficiently lower moisture content, the one-third reduction in successive layer depths may not be necessary. Actually, the maximum quantity of grain can be dried in a bin when each layer contains only the quantity that can be dried each day (daily fills); but this usually requires extra work, because the loading auger must be moved more often to fill each bin every day and each layer must be leveled in order to dry. When loading and leveling, try to spread any trash or foreign material uniformly so as not to block the air flow.
If your fan is smaller than that recommended in Table 23, the depth of each layer of wet grain must be reduced to maintain the necessary air flow to prevent deterioration.
There should never be more wet grain in a bin than can be dried in four days. The depth of wet grain remaining in a bin can be determined by probing the grain with a ¼ in. smooth steel rod. A definite change in the resistance to the penetration of the rod will be noted when the end enters the dry grain. Caution: Each layer must be leveled before drying for all grain. A mechanical grain leveler is very useful for this purpose.
Drying Heat
Layer drying is accomplished with relatively little heat. During warm sunny days only unheated natural air is used. During periods of high humidity and at night, a small quantity of heat is used to achieve the desired rate of drying. The quantity of heat used affects both the rate of drying and the final moisture content because the lower layers continue to dry until they reach equilibrium with the drying air. This final moisture can be estimated by referring to Table 26. The ideal relative humidity for layer drying is 50 percent to 60 percent to achieve a good storage moisture content. This ideal 50 percent to 60 percent drying humidity is achieved by using natural, unheated air during fair days and a 15° to 20°F heat rise at night and during cool, damp days. A 15° heat rise means that it is 15° warmer under the bin than outside. Do not check the temperature in the top of the bin, because evaporative cooling occurs as the air passes up through the wet grain and picks up moisture.
Higher heat will speed drying but will overdry the grain. The amount of overdrying can be estimated by referring to Table 26 after determining the relative humidity of the drying air by the following rule: Each 20°F of heat rise will cut the relative humidity in half. Example: A 20°F heat rise during a damp night with 100 percent relative humidity (rh) gives 50 percent relative humidity under the bin (100 percent rh @ 60° + 20° = 50 percent rh @ 80°).
As the bin is filled, the added grain depth increases the static pressure the fan operates against. This increased static pressure reduces the total air flow, causing an increase in the heat rise or temperature under the bin. The increased temperatures will further lower the humidity, causing the bottom layers to continue to dry and delaying the drying of the top layer. To maintain the original moisture content in the lower layers of grain, the heat input (Btu) must be reduced as the bin is filled.
The heat is adjusted by varying the gas pressure on the burner and/or changing the orifice (nozzle) size that the gas passes through. Some drying fans and heaters have a "high and low" valve; this valve should always be in the low position for layer drying.
Tips for Drying and Storage
- Purchase adequate drying equipment.
- Check bins for holes or gaps that should be sealed before the grain is loaded.
- Thoroughly clean the grain bin to remove leftover grain and debris.
- Apply empty bin treatment or fumigate as necessary to eliminate any leftover insects.
- Clean grain to remove excessive fine particles if necessary.
- Dry grain to recommended moisture content before storage or use layer method to dry grain.
- Apply a grain protectant insecticide as grain is loaded into bin. If grain is to be dried in the bin do not apply a protectant insecticide because it will break down with heating. In this case it will be necessary to transfer the grain out of the bin, and treat with a protectant as it is loaded back in.
- Start the fan as soon as the floor is covered with grain.
- Keep all exhaust hatches open when fan is operating.
- Don't put more wet grain in the bin than the amount that can be dried in four days.
- Level each layer of grain, including the uppermost layer of grain.
- Don't let high-moisture grain heat from respiration.
- Dry in both bins at the same time for maximum drying speed when two bins are equipped with only one fan.
- Begin to aerate to cool grain as soon as possible after drying.
- Continue to cool at every opportunity when the weather is dry and cooler than the grain until grain temperature is below 55°F.
- Continue to aerate periodically during storage.
- Do not freeze grain.
- Do not aerate during warm humid weather after the grain is cold. This will cause moisture to condense in the grain mass.
- Probe and inspect grain during storage for heating, damage, and insects. Sample every 20 days in spring, summer and fall; every 30 days in winter. Take at least five samples from each bin. If insect infestations are found, grain should be used as quickly as possible (on-farm feed) or fumigated to allow for continued storage.
Consult the Georgia Pest Management Handbook or the Alabama Cooperative Extension Service IPM-330, Stored Grain Insect Control Recommendations for specific insecticide recommendations.
MILLING AND BAKING QUALITY OF SOFT RED WINTER WHEAT
Soft red winter wheat grown in the southeastern United States is suitable for a wide variety of products. The flour of soft red wheat is finely granulated, low in protein, and has low absorption (water holding capacity) with weak gluten features, making it uniquely suited for a variety of products. In the Southeast, soft wheats primarily are used for all-purpose flour. Soft red wheat flours are preferred in cookies, cakes, crackers, pie crusts, donuts, biscuits, flat breads, batters, gravies, soups, ice cream cones and starches. At least eight soft wheat flour types are used, which includes three cake flours, two cookie flours, and cracker, pastry and thickener flours.
Crackers and flat breads rely on protein strength for structure. The balance between protein strength and protein quantity is very important in determining end-product acceptability. Cultivars that have the stronger protein type are better suited for cracker production. The increased number of major Southern soft wheat cultivars has broadened the variability of wheat protein quality or strength.
Soft wheat quality evaluation is separated into two distinct processing steps: milling and baking (Table 27). Milling quality criteria include bran endosperm separation, kernel hardness and flour yield. Flour yield is the most important trait for milling quality evaluation. One hundred lb of wheat should yield more than 70 lb of white flour. Baking quality refers to the potential of flour to contribute to the acceptability of a baked product and is measured by flour protein quality and quantity, flour viscosity, ash and flour color. Generally, good quality soft wheats should have high flour yield, low protein content, high softness equivalent (soft kernel) and low water absorption (AWRC). Soft texture kernels result in high flour yield. Low water absorption improves flour-baking quality. Cookie diameter gives an evaluation of the actual baking performance of flour.
Table 27. Soft wheat quality traits for cultivars grown in the Southeast
Cultivar |
Quality Scores1
|
Test Weight lbs/bu
|
Soft Equiv.
|
% Flour Yield
|
% Flour Protein
|
AWRC
|
Cookie Diameter (cm)
|
|
Milling
|
Baking
|
|||||||
Florida 302 |
104A
|
97B
|
56
|
57
|
71
|
8.8
|
57
|
16.8
|
Saluda |
100A
|
100A
|
58
|
57
|
69
|
8.7
|
61
|
17.1
|
Coker 9835 |
107A
|
102A
|
59
|
60
|
71
|
7.7
|
63
|
17.5
|
GA Dozier |
102A
|
101A
|
59
|
54
|
70
|
8.9
|
58
|
17.1
|
1 Higher or lower than the standard check. Letter ranking from A to F (excellent to very poor).
Environmental conditions greatly influence milling and baking quality, especially kernel hardness which results in lower break flour yield, coarser flour, higher protein content and lower water absorption. Field sprouting of wheat, which occasionally occurs in the Southeast, can be a serious problem for milling and baking quality. Sprouting causes particular problems for wheat that would otherwise be used for cracker production. Sprouted grain has enzyme activity that interferes with the fermentation process which makes it unsuited to cracker dough batch development.
Flour mills in the Southeast grind millions of bushels of wheat into flour annually. Millers have increased their purchasing of southeastern wheat due to the improved quality of new cultivars. Millers purchase wheat with a protein content of 11 percent or less and a test weight of 58 lb/bu or above. The quality of wheat grown in the Southeast is dramatically influenced by the season and cultivar. Variation for quality traits can exist within a state due to soil type, agronomic management and cultivar selection. Diseases of wheat also affect milling and baking quality of soft red wheat. In one test, the most susceptible variety to powdery mildew had the lowest protein content and the best quality when disease levels were high. However, reductions in wheat quality often occur in response to severe leaf rust infection. Protein content often increases as disease severity increases.
Time and rate of nitrogen (N) fertilization influences milling and baking quality. Fall N application above 30 lb/acre has a detrimental effect on quality traits. Spring N rates reduced baking quality by increasing protein content. The trend toward rates above 80 lb/acre may result in higher protein content, which would lower baking quality. Nitrogen applications at the recommended rate of 60 to 80 lb/acre in the spring do not result in any adverse effects on milling and baking quality.
In summary, cultivar selection can play an important role in milling and baking quality. Wheat diseases in the Southeast can influence quality if they reduce test weight. The recommended N rates of 60 to 80 lb/N in the spring should not alter milling and baking quality.
PRODUCTION COSTS AND MARKETING ALTERNATIVES
Know All Your Costs
Outlays for fertilizer, seed and fuel are usually considered major cost items for growing wheat. But there are numerous small cash outlays plus allowances for items such as equipment depreciation, farm overhead, management and profit. It is essential to know all these costs for any enterprise analysis.
The first step in wheat production is to estimate the costs per acre, and then get these costs on a per bushel basis. The worksheet in Table 28 is designed for this purpose. Costs estimates are given for two systems of wheat production 1) one that includes those inputs necessary to obtain an average wheat yield of 40 bushels/acre, and 2) a system of intensive management to achieve a yield of 75 bushels/acre. These latter input levels will not ensure higher production levels but are a prerequisite to a more intensive management system to attempt those yield levels.
The production costs are not precise. They are intended to provide guidelines to help each producer estimate his own costs for the production system being considered. Several cost items included in the worksheet are non-cash items — machinery depreciation, management, family labor and land costs (if the land is owned). A wheat grower can usually cover cash costs with wheat sales. But these non-cash items are included to point out the sale price needed to cover all costs over a period of time.
Table 28. Wheat for grain cost worksheet
Cost Item
|
Unit
|
Price/Unit
|
Conventional System (40 bu/acre)
|
Intensive System (75 bu/acre)
|
Cost
|
||
No. Units
|
Cost/Acre
|
No. Units
|
Cost/Acre
|
||||
NON-CASH COSTS | |||||||
Machinery Depreciation |
|
||||||
Preharvest |
Acre
|
13.00
|
1.00
|
13.00
|
1.00
|
13.00
|
|
Harvest |
Acre
|
17.00
|
1.00
|
17.00
|
1.25
|
21.25
|
|
Unpaid Family Labor |
Hour
|
7.00
|
2.25
|
15.75
|
3.00
|
21.00
|
|
Management |
Acre
|
5.00
|
1.00
|
5.00
|
1.25
|
6.25
|
|
Land Rental Value |
Acre
|
40.00
|
1.00
|
40.00
|
1.00
|
40.00
|
|
Total Non-cash Costs |
|
|
|
|
|
|
|
Per Acre |
|
|
|
$90.75
|
|
$101.50
|
|
Per Bushel |
|
|
|
$2.27
|
|
$1.35
|
|
CASH COSTS |
|||||||
Seed |
Lb.
|
10.00
|
1.50
|
15.00
|
2.50
|
25.00
|
|
Lime |
Ton
|
24.00
|
0.25
|
6.00
|
0.25
|
6.00
|
|
Fertilizer |
|
||||||
Nitrogen |
Lb.
|
0.30
|
80.00
|
24.00
|
120.00
|
36.00
|
|
Phosphate (P2O5) |
Lb.
|
0.22
|
40.00
|
8.80
|
50.00
|
11.00
|
|
Potash (K2O) |
Lb.
|
0.13
|
40.00
|
5.20
|
60.00
|
7.80
|
|
Herbicides |
Acre
|
2.00
|
1.00
|
2.00
|
1.00
|
2.00
|
|
Machinery – Preharvest |
|
|
|
|
|
|
|
Fuel |
Gallon
|
0.95
|
3.00
|
$2.85
|
4.00
|
$3.80
|
|
Repairs and Maintenance |
Acre
|
8.00
|
1.00
|
8.00
|
1.20
|
9.60
|
|
Machinery – Harvest |
|
|
|
|
|
|
|
Fuel |
Gallon
|
0.95
|
2.00
|
1.90
|
3.00
|
2.85
|
|
Repairs & Maintenance |
Acre
|
12.00
|
1.00
|
12.00
|
1.20
|
14.40
|
|
Mach., Taxes & Insurance |
Acre
|
3.00
|
1.00
|
3.00
|
1.20
|
3.60
|
|
Fungicides |
Acre
|
9.00
|
1.00
|
9.00
|
1.00
|
9.00
|
|
Insecticides |
Acre
|
6.00
|
.00
|
.00
|
1.00
|
6.00
|
|
Seed Treatment |
Acre
|
0.50
|
.00
|
.00
|
2.50
|
1.25
|
|
Overhead |
Dollar
|
0.05
|
108.00
|
5.40
|
148.00
|
7.40
|
|
Hired Labor |
Hour
|
7.00
|
.00
|
.00
|
.00
|
.00
|
|
Interest on Production Capital |
Dollar
|
0.10
|
103.15
|
5.16
|
145.70
|
7.29
|
|
Drying – 2 Points |
Bushel
|
0.08
|
36.05
|
2.88
|
77.20
|
6.18
|
|
Total Cash Costs |
|
|
|
|
|
|
|
Per Acre |
|
|
|
$111.19
|
|
$159.17
|
|
Per Bushel |
|
|
|
$2.78
|
|
$2.12
|
|
TOTAL ALL COSTS | |||||||
Per Acre |
|
|
|
$201.94
|
|
$260.67
|
|
Per Bushel |
|
|
|
$5.05
|
|
$3.48
|
|
EXPECTED SALE PRICE |
|
||||||
Prospect Per Unit |
|
Single Versus Double Crop
The cost estimates provided are based on wheat being grown in a single rotation. Most of the increase in wheat acreage in the Southeast has occurred in a doublecropping system with soybeans. A primary economic advantage of doublecropping is to allocate the fixed costs of the machinery over two crops.
Because wheat is usually harvested in May or June in the Southeast, second crop soybeans usually experience a decrease in yield resulting from their being planted later than usual. In effect, the wheat-soybean rotation results in lower overhead costs per crop but has two cash flow periods — in June (wheat) and in the fall (soybeans).
Wheat production costs should first be estimated as a single crop. If a doublecropping scheme is used, consider the advantages (cash flow and using equipment on two crops) versus the disadvantages of any reduced soybean yield.
Marketing Alternatives
Current government policy mandates a market-oriented system of price determination. As a result, southeastern wheat producers have a greater price risk in deciding how much to produce as well as when, where and how to establish a price.
The increased importance of foreign markets on wheat prices complicates the marketing problem. Changes in the value of the dollar against foreign currencies, combined with uncertainties in global weather and political situations, make marketing risky. Farmers should react to these conditions by utilizing marketing practices that reduce price risk and increase the probabilities of selling their crop at profitable prices.
There are many marketing alternatives available to southeastern wheat farmers. These alternatives allow for the pricing of a crop up to one year prior to harvest to more than one year after harvest. A prudent strategy is to reduce price risk by making sales whenever profitable prices are offered.
Several marketing alternatives are available in regard to delivery pricing:
- The Cash Market - This is the most important market for grain grown in the Southeast. Delivery can occur at harvest or after the crop has been in storage for some time. Price is established at the time of delivery. A large portion of southeastern wheat is sold at harvest time. Harvest sales are attractive because payment is made at the time of delivery. Unfortunately, harvest sales often occur at the seasonal low price. Sales direct from the combine often result in quality discounts for high moisture and excess foreign matter that further reduces the price. Also, harvest time sales may be a poor use of labor and equipment due to waiting lines at buying points.
- Storage and Later Sales - As commodity prices can make wide swings from harvest until later in the marketing year, storage combined with later sales attempts to take advantage of price fluctuations. However, storage does not guarantee a high or profitable selling price, but it does allow greater flexibility in production and marketing. Each situation is different, but generally the total monthly costs for storage will range between 6 and 10 cents per bushel for wheat.
- Cash Forward Contracts - Cash forward contracting or "booking" is an agreement between a seller and a buyer for a specified quantity and quality of crop at a specified price and delivery period. The buyer is usually an elevator but may be a miller, feed mixer or even another farmer. Cash contracts work because they offer advantages to both parties. The grower gains by shifting price risk to the buyer as well as assuring a buyer ahead of actual delivery time. Cash contracts limit downside price risk.
Contracts present two main disadvantages for the seller. Upside price gains are not possible and contracts may magnify production risk. For example, if a farmer fails to produce enough crop to meet a contract, he may have to buy wheat to meet the contract.
Forward Pricing Alternatives
Hedging in the Futures Market - Hedging is not a viable alternative for all farmers; however, knowledge of the futures market is needed to understand how local commercial buyers operate. The bids offered by local buyers are tied directly to the futures market. The futures markets are markets at which prices are established for the future delivery of commodities. They are very active markets with many buyers and sellers in which large volumes of contacts (representing actual commodities) are traded.
The futures market allows a producer to lock in an approximate and predictable price buy, not an exact price. Prices in the local cash market and in the futures market tend to move in the same direction and by about the same amount. However, they usually will not be identical, and they will not always parallel each other. Local cash prices represent the local supply and demand situation influenced heavily by the greater national and even global situation. On the other hand, futures prices reflect the collective interpretation of all available information on the domestic and worldwide supply and demand situation by all the market participants.
The difference between the local cash price and the futures market price is called basis. The basis is reasonably predictable and certainly more so than the actual cash or futures price. The risk of predicting the wheat basis is much less than the risk of predicting cash wheat prices. The futures market permits the hedger to transfer the risk of predicting the cash price for the lesser risk of predicting the basis.
Hedging has several advantages. Hedging reduces price risk and minimizes the potential for loss as a result of unfavorable price movements. Hedging allows for continued ownership of the crop and allows flexibility in pricing decisions. The potential for basis gains exists in a hedge. The hedged commodity possesses a more secure value for use as collateral.
Hedging also has potential disadvantages. Hedging requires greater management effort than other market alternatives. Because a hedged commodity is still owned by the hedger, ownership risks are still present. Hedging also has potential short-term negative cash flow implications as additional margin deposits are required if the price moves against the futures market position. Hedging has proven through time to be an effective marketing tool for shifting price risk to those willing to accept it.
Delayed Pricing
Delayed pricing alternatives involve the use of contracts under which a commodity is delivered to a buyer, title changes hands, and the seller agrees to "price" the commodity during a future time period. The comments made earlier about the use of well-written contracts apply here as well. In fact, even more so, for with these sales alternatives the seller surrenders possession and title to the commodity before price is determined and full payment is received.
- Price Later Contracts (PLCs) - PLCs are contracts under which a commodity is delivered to the buyer. The title changes and the seller may accept the price offered by the buyer on any given day within some specified time period. Usually buyers that offer PLCs immediately hedge their purchase in the futures market to guard against price rises. By hedging, the buyer does not need to keep the wheat on hand. The wheat can be moved and the buyer is assured of funds to pay the seller when the pricing decision is made.
PLCs are most commonly signed during harvest and offer several potential advantages to the seller. The seller can gain from the typical seasonal price improvement and basis appreciation that often occurs following harvest without having to incur the risks and costs of maintaining a stored commodity. Storage risks and costs are transferred to the buyer. These contracts also allow for flexibility in planning income for tax purposes. This purpose can be accomplished by signing a price later contract and avoiding storage obligations.
In view of many observers, the greatest disadvantage to PLCs is the potential default by the buyer. Legally, the seller becomes an unsecured creditor to the buyer when a PLC is signed. In the event of bankruptcy by the buyer, the seller holds an interest in the remaining assets of the business inferior to that of secured creditors.
- Basis Contracts (BCs) - Basis contract are similar in many respects to PLCs. They differ in the manner in which the price is determined. Under a BC, the commodity is delivered to the buyer, title changes hands, and the seller selects a futures market contract month near the date of expected sales upon which the price will be based. The buyer assigns a "basis" to the futures contract. The seller agrees to "price" the contract before the futures contract matures. A variation on this is to assign a basis to each futures contract for the crop year. The seller prices at his discretion, and the current near-month contract is used as the base. The buyers hedge the contract in the same way as explained above.
- Pricing With Commodity Options - The options market provides producers with the opportunity to sell or buy a commodity at a specified price. Just as one may purchase the right from an insurance firm to collect on a policy in case the building burns, one can purchase the right to sell one's commodities at a specific price if market prices go below the specified price.
Purchasers of options have the opportunity but not the obligation to exercise their agreement. For instance, if you desire to buy the right to sell wheat for $3.00 per bushel, the commodity options market provides the opportunity. By paying the market-determined premium, you can then collect on the option if prices are below $3.00 per bushel when the wheat is sold. If prices are higher than $3.00 per bushel, the crop can be sold for the higher price and the cost of the premium is absorbed.
There are actually two basic types of commodity options: a call option and a put option. The call option gives the holder the right, but not the obligation, to buy the underlying commodity from the option writer at a specified price on or before the option's expiration date. The put option gives the holder the right, but not the obligation, to sell the underlying commodity to the option writer at a specified price on or before the option's expiration date.
The "specified price" is the price at which the underlying commodity can be exchanged and is fixed for any given option. Several options with different strike prices are traded during any period of time.
Options on agricultural commodities have futures contracts as the underlying commodity. Futures contracts have a definite predetermined maturity date during the delivery month. Options will also have a date at which they mature and expire. For example, a $3.00 July wheat put option is an option to sell one July wheat futures contract at $3.00 on any business day until late in June at which time the option expires. Trading the options will not be conducted during the delivery month. Upon expiration, the option becomes worthless.
The advantages of using put options include: never receiving less than the strike price less basis and transaction cost, receiving more than the cash price in a falling market, receiving more than a hedger in a rising market, the ability to sell at a higher price at a later date, and the ability to use with immediate or future delivery. Disadvantages of options include: the cost of the option, the seller will never receive as much as a cash sale in a rising market, he will receive less than a hedger in a falling market and in a steady market, and options will be more expensive than hedging or holding cash grain.
Summary
Southeastern wheat producers have several production and market alternatives available. The successful producer will determine a price objective, develop a marketing strategy, evaluate one's ability and willingness to take price risks, analyze all available market alternatives, determine which combination has the greatest chance of success and then make and execute the decision.
WHEAT IN SWINE DIETS
The primary sources of energy in swine diets (rations) are cereal grains. Traditionally, corn has been the mainstay of most swine feeding programs. However, during times of high corn prices and/or low wheat prices, wheat can be an attractive alternative to corn for swine diets. Research indicates that wheat can be efficiently utilized by swine of all ages; however, when using wheat to replace corn or other cereal grains in swine diets, considerations must be given to the nutrient composition, method of processing, quality, and relative value compared with the price of other grains.
Nutrient Composition
The comparative composition of soft red winter wheat and corn, as well as other feed ingredients, is shown in Table 29. Wheat is higher than corn in crude protein but, more importantly, is higher in lysine. Some of the amino acids including lysine are termed "essential amino acids," and must be present in the diet for normal growth and function of pigs and other simple stomached animals. Amino acids are the building blocks of protein. The protein of corn, wheat or other cereal grains is deficient in certain of these essential amino acids and must be fortified with a protein supplement such as soybean meal. Lysine is especially important because it is the first limiting essential amino acid in a grain-soybean meal based diet. This means that if a diet is formulated to supply the correct amount of lysine, then, generally, the levels of the other essential amino acids will be adequate. Therefore, lysine content is an important consideration when comparing cereal grains.
Wheat contains less fat than corn, but is similar to corn in metabolizable energy content (Table 29). Phosphorus content of wheat is slightly higher than that usually found in corn, and phosphorus in wheat is more available (digestible) to pigs.
Table 29. Typical analyses of wheat and other feedstuffsa
Feedstuff |
Metabolizable Energyb, kcal/lb
|
Crude Protein %
|
Lysine %
|
Calcium %
|
Phosphorus %
|
Wheat, soft red |
1500
|
11.5
|
.32
|
.05
|
.36
|
Corn |
1550
|
8.5
|
.25
|
.02
|
.28
|
Grain sorghum |
1450
|
9.5
|
.23
|
.03
|
.28
|
Oats |
1200
|
11.5
|
.40
|
.05
|
.34
|
Soybean meal (44%) |
1450
|
44.0
|
2.90
|
.30
|
.65
|
Soybean meal (48%) |
1500
|
48.5
|
3.10
|
.25
|
.60
|
Dicalcium phosphate |
–
|
–
|
–
|
22.00
|
18.50
|
Defluorinated phosphate |
–
|
–
|
–
|
32.00
|
18.00
|
Mono-dicalcium phosphate |
–
|
–
|
–
|
18.00
|
21.00
|
Limestone, ground (calcium carbonate) |
–
|
–
|
–
|
38.00
|
.04
|
a Values given are typical for feedstuffs used in the southeastern United States (expressed on an as-fed basis).
b Relative energy (feeding) value compared with corn = 100: wheat = 100, grain sorghum = 95, oats = 80.
Results of Feeding Trials
Research designed to evaluate soft red winter wheat in swine diets has been conducted in Kentucky, Georgia and Florida. A summary of this research indicates that a wheat-soybean meal diet is essentially equal in feeding value to a corn-soybean meal diet as measured by average daily gain or feed conversion of growing-finishing swine.
Use of Wheat in Swine Diets
Even though wheat contains more protein than corn, diets should be formulated based on lysine and not protein. The analyzed crude protein content of a wheat-based diet will usually be higher than a corn-based diet when both diets contain equal content of lysine. If the diets containing wheat were formulated to meet the crude protein requirement, lysine level would be inadequate to support maximum performance in swine. Lysine analysis, however, is expensive, but can be estimated from the protein content by a competent swine nutritionist.
In general, the higher lysine content of wheat usually can result in a savings of 50 lb of soybean meal (48 percent) per ton of mixed diet over a comparable corn-based diet. Examples of growing and finishing diets using soft red wheat are shown in Table 30.
Producers using a commercially available complete protein-vitamin-mineral supplement should consider wheat equal to corn and substitute wheat for corn on an equal weight basis when mixing swine diets. To decrease the amount of these complete supplements to take advantage of the higher lysine (protein) content of wheat would dilute the essential minerals and vitamins that these supplements also provide to the diet. This dilution could lead to inadequate mineral and vitamin levels in the diet, resulting in poor pig performance. Many problems associated with feeding wheat can be the result of improper protein supplementation.
Table 30. Example of swine growing finishing diets using soft red wheat or corn (lb/ton)
Grower (50 to 120 lb) | Finisher (120 to 240 lb) | |||
Corn | Wheat | Corn | Wheat | |
Ground wheat |
–
|
1,600
|
–
|
1,700
|
Ground corn |
1,550
|
–
|
1650
|
–
|
Soybean meal (48%) |
400
|
350
|
300
|
250
|
Base mixa: | ||||
Dicalcium phosphateb |
20
|
20
|
20
|
20
|
Ground limestone |
20
|
20
|
20
|
20
|
Salt |
7
|
7
|
7
|
7
|
Vitamin-trace mineral premixc |
3
|
3
|
3
|
3
|
Total |
2,000
|
2,000
|
2,000
|
2,000
|
Calculated composition (as fed basis): | ||||
Crude protein,% |
16.4
|
17.6
|
14.4
|
15.8
|
Lysine,% |
0.80
|
0.80
|
0.68
|
0.66
|
Calcium,% |
0.66
|
0.70
|
0.66
|
0.70
|
Phosphorus,% |
0.52
|
0.56
|
0.50
|
0.54
|
a A complete mineral-vitamin premix or a complete mineral premix and separate vitamin premix may be used instead of the suggested base mix. Follow manufacturer’s guidelines. If needed, increase or decrease the grain portion of the diet so that the total would equal 2,000 lb.
b Deflorinated phosphate or mono-dicalcium phosphate, if available, may be substituted for dicalcium phosphate. If a substitution is made, the diets will need to be reformulated since these products contain different calcium and phosphorus levels than does dicalcium phosphate (see Table 29).
c Commercially available swine vitamin and trace mineral premix(s).
Wheat Processing
Processing wheat for efficient use in swine diets is very important. Best results in university feeding trials were obtained when wheat was coarsely ground or lightly crushed (lightly rolled). Hammer mill screen size of 5/16 to ¼ in. and perhaps reduced hammer mill rpms should provide an acceptable grind. However, the wheat should be ground such that there are no whole kernels. Finely ground wheat is not desirable because it easily absorbs moisture from the atmosphere and the pig's own saliva, which can result in feed spoilage and reduce feed intake. This absorption of moisture and subsequent feed spoilage is a particular problem in hot, humid areas such as the southeastern United States. Additionally, diets with finely ground wheat can bridge and not flow well in self-feeders. These problems may still occur even with coarsely ground wheat in the ration. Under these conditions, mixing wheat with ground corn is advisable.
Low Test Weight, Sprout Damaged and Contaminated Wheat
Wheat of low test weight is usually discounted or "docked" when sold for human consumption and, if the test weight is too low, it cannot be sold for human consumption at any price. This low test weight is usually due to shrunken and shriveled kernels or sprout damage in the kernels. In most instances, this wheat can be included in swine diets without affecting performance. In fact, wheat kernels that are shrunken and shriveled are usually higher in crude protein and lysine than normal wheat kernels. However, unless it can be analyzed for lysine content, it is suggested that this wheat be used in swine diets much like wheat of higher test weight. Wheat of low test weight should be purchased and used in the diet on a weight basis rather than on a volume basis. Wheat of very low weight (below 52 lb/bu) will usually have a lower feeding value than heavier wheat for pigs mainly due to higher fiber content. Fiber is not well utilized by swine, and thus the metabolizable energy (ME) content will be reduced. In one study, the feeding (energy) value of 51 lb/bu wheat was 7 percent lower and 45 lb/bu wheat was 10 percent lower than that of 59 lb/bu wheat.
Sprout damaged wheat has sprouted in the head before harvest and usually has a decreased test weight. Feeding tests show that sprout damage had little effect on the feeding value of soft winter wheat for growing-finishing swine. Therefore, sprout damaged wheat can be effectively used in swine diets the same as undamaged wheat provided that the grain is dry and mold free. If in doubt about the acceptability of sprout damaged wheat, it should be test fed to a small group of pigs before purchasing large quantities. Sprout damaged wheat should be mixed with undamaged wheat or corn if there are acceptability problems.
Wheat contaminated with various foreign materials such as rye, mustard seed, wild garlic and/or wild onion can be fed to swine with few problems. Wheat with extreme contamination, especially with wild garlic and/or onion, may be unpalatable to pigs and may reduce feed intake. Again, if in doubt about the acceptability of this wheat, it should be test fed to a small group of pigs before purchasing large quantities. Contaminated wheat should be mixed with uncontaminated wheat or corn if there are acceptability problems.
Relative Value of Wheat
The relative value of wheat compared with corn at varying corn and soybean meal prices is shown in Table 31. Because of its higher lysine content than corn, the relative price of wheat also is dependent upon the price of soybean meal or other protein (lysine) sources. The higher the price of soybean meal, the higher the value of wheat relative to corn.
As an example, when soybean meal is $200/ton and corn is $2.50/bu, wheat would have an equivalent value of $2.65/bu. Therefore, wheat purchased at less than $2.66/bu would result in more economical gains. On a bushel basis, wheat would be worth more than corn because a bushel of wheat is heavier than a bushel of corn (58 to 60 lb vs. 56 lb). However, for producers who mix their own diets using a complete protein-vitamin-mineral supplement, wheat would only be worth the same price as corn (pound for pound bases) because wheat should only replace corn on a pound for pound basis when using complete supplements.
Table 31. Maximum price that can be paid for soft red wheat to replace corna
Soybean meal (48%) $/ton |
Corn $/bu
|
|||||
2.00
|
2.25
|
2.50
|
2.75
|
3.00
|
3.50
|
|
140 |
2.11
|
2.36
|
2.61
|
2.86
|
3.11
|
3.60
|
160 |
2.13
|
2.38
|
2.63
|
2.87
|
3.12
|
3.62
|
180 |
2.14
|
2.39
|
2.64
|
2.89
|
3.14
|
3.64
|
200 |
2.16
|
2.41
|
2.66
|
2.91
|
3.16
|
3.66
|
220 |
2.18
|
2.43
|
2.68
|
2.93
|
3.17
|
3.67
|
240 |
2.20
|
2.45
|
2.70
|
2.94
|
3.19
|
3.69
|
260 |
2.21
|
2.46
|
2.71
|
2.96
|
3.21
|
3.71
|
a Based on wheat having lysine content = 0.32%, relative energy = 99% of corn and test weight = 58 lb/bu.
Summary
- Wheat may be used as a partial or sole grain source in mixed diets for all classes of swine.
- Diets containing wheat should be balanced for lysine instead of crude protein. A wheat-soybean meal diet will be higher in crude protein content than comparable corn-soybean meal diet.
- Wheat contains more lysine than corn, thus those producers who mix their own diets using a soybean meal-premix program can save 50 lb of soybean meal (48 percent) per ton of mixed diet.
- Those producers who mix their own diets using a complete protein-vitamin-mineral supplement should think of wheat as a corn replacement only and not a partial protein supplement replacement as well.
- Wheat should be coarsely ground or rolled for use in swine diets.
- Wheat is worth up to 6 percent more than the price of corn on an equal weight basis for those who can take advantage of wheat's higher lysine content. Otherwise, wheat is only worth as much as corn.
SMALL GRAINS FOR NON-GRAIN USES
Forages
Rye, wheat and oats are widely used in winter forage programs for cattle. Small grains grown alone or in combination with ryegrass and/or a winter annual clover can provide grazing in late fall and spring. These crops produce excellent quality forage that is very digestible (65 percent +) and has a high protein content (12 percent to 18 percent). Forage quality is highest when the plants are growing vegetatively and declines as the plants start heading. Small grain crops also can be grazed and/or cut for silage.
Crop Selection
Rye is more frequently planted for grazing than the other small grains. It is more cold tolerant and usually produces more late fall and winter grazing. Rye matures earlier in spring than wheat or oats and has a shorter spring grazing season.
Wheat and oats have similar growing seasons. These crops produce less fall grazing, but because they mature later in spring, they can be grazed two to three weeks later than rye. Oats are less cold tolerant than rye or wheat and may winter kill during severe winters. Wheat and oats produce high yields (6 to 8 tons/acre) of good quality silage (60 percent to 62 percent). Small grain forage yields are annually reported in state small grains performance bulletins. Wheat and oats are dependable silage crops that fit into many doublecropping programs.
Ryegrass is an excellent crop to plant in combination with a small grain for grazing or silage. Ryegrass matures later in spring than the small grains and produces most of its growth in spring when the small grains are maturing. When ryegrass is planted in mixtures with the faster growing small grains, the small ryegrass seedlings may be severely shaded in fall unless the pasture is lightly grazed to retard competition.
Seeding Dates
Plant small grains, ryegrass, and annual clovers in the fall when soil moisture is favorable for seed germination and plant growth. Seed in late August in the mountains, early September in the Piedmont, and late September to early October in the Coastal Plain. Seeding earlier than these dates may result in increased insect and disease problems due to the higher temperatures.
Seeding Rates
Small grains planted for forage are seeded at higher rates than those planted for grain production (Table 32). Higher seeding rates provide more forage in fall and early winter.
Table 32. Seeding rates per acre for annual grasses
Crop
|
Pure Stands
|
Mixtures
|
Rye
|
2-3 bu
|
2 bu
|
Wheat
|
2-3 bu
|
2 bu
|
Oats
|
3-4 bu
|
3 bu
|
Ryegrass
|
30-40 lbs
|
20 lbs
|
Planting
Small grains for forage are best established on a clean, well-prepared seedbed. Seed should be planted to a uniform depth of 1 to 1½ in. Grain drills that are properly adjusted and operated can provide uniform planting depths. Broadcasting seed and disking or dragging to cover the seed usually produces less uniform stands due to less precise seed placement. Increase seeding rates 20 percent when seed is broadcast.
When seeding ryegrass or annual clovers in combination with a small grain, be careful not to cover the ryegrass or clover with more than ¼ to ½ in. of soil. Broadcasting the small-seeded crop before drilling the small grain seed usually provides adequate coverage for clover or ryegrass.
Fertilization
A good fertilization program is essential for high yields of good quality forage for grazing or silage. Conduct a soil test several weeks prior to seeding and apply dolomitic limestone if the soil pH is below 5.8.
Phosphorus and potash, at the rates indicated by soil test results, should be applied and incorporated before seeding. Small grains managed for grazing can utilize up to 150 pounds of nitrogen per acre. Three equal applications — fall, midwinter, and spring — are usually the most efficient way to apply nitrogen in an intensively managed system. The fall nitrogen application may be made at planting or after the seedlings have germinated and started to grow.
Grazing Mixtures
Small grains may be planted in pure stands or grown in mixtures with ryegrass and/or a winter annual clover. Ryegrass grows vigorously in spring and, when used in mixture, extends the grazing season. Crimson and arrowleaf clover also are frequently used in combination with a small grain and ryegrass for winter grazing.
During midwinter, growing conditions may be unfavorable. Avoid severe overgrazing which can weaken stands and reduce spring forage production. Small grains grow rapidly in spring and forage quality declines as the plants start reproductive growth. Increase grazing in spring to improve utilization. If the crop is being managed for spring grain production, remove cattle before the jointing stage.
Silage
Wheat and oats are the most commonly used small grains for silage. Agronomic practices for silage production are similar to practices for grazing.
The harvest date of small grains for silage is very important. As plants develop beyond the boot stage and into early grain fill, the protein and energy levels drop while the fiber level rapidly increases. Although there is a general increase in dry matter yield as the crop matures, the increased yield is more than offset by the reduction in forage quality. Consequently, the best time to cut small grains for silage is in the boot to early heading stage.
Small grains reach the heading stage usually in late March to late April depending on planting date and type of grain and varietal maturity. Usually, there can be seven to 10 days' difference in heading date between the earliest and latest maturing varieties.
Small grains cut earlier than the soft dough stage will require wilting in order to make high quality silage. The length of wilting time required will vary, depending on the drying conditions and stage of maturity. Approximately 35 percent to 40 percent dry matter is desirable for ensiling. Cutting length should be 3/8 to 2 in. for good ensiling. Silage should be packed tightly to exclude as much air as possible. As silage becomes drier, it is more difficult to maintain the optimum length of cut and ensiling. To maintain good silage production, consideration should be given to the amount of acreage to be cut and the amount of time needed to cut the crop within the proper maturity stage.
Hay Production
Small grains can provide a good source of hay when properly cut, cured, and baled. For best yields and quality, small grains should be cut between late boot and early heading stages. Agronomic practices used in grain production are suitable for hay production. It is frequently difficult to make good small grain hay in the Southeast due to poor drying conditions.
Straw Utilization
Straw is commonly burned after harvest in the Southeast to prepare soil for planting a summer doublecrop. Burning straw releases nutrients in the straw and makes them readily available to the next crop. Burning also reduces interference with tillage equipment during seedbed preparation for the next crop. However, burning straw has little value in reducing weeds, insects, and most pathogens and may increase the potential for soil erosion and loss of soil moisture through evaporation. Burnt straw also is highly attractive to lesser cornstalk borers and may increase damage by this insect to seedlings of the following summer crop, especially soybeans. Burning also contributes to regional air pollution and can cause a smoke hazard on nearby roads.
Straw can be harvested and used as animal feed, bedding, landscaping or mulch. If straw is to be sold or used off farm, then the straw is usually baled for easier handling. Straw residue after grain harvest is left in windrows behind the combine or teddered into windrows to facilitate baling into small rectangle bales. Generally, bales are 40 to 50 lb each. Large round or square bales weighing in excess of 700 lb are more efficient for on-farm uses as feed.
Small grain varieties can vary considerably in straw production. Examples of straw performance of wheat and barley are shown in tables 33 and 34. Production can vary from as little as 0.5 tons to over 2.5 tons. Note the variability in yield and height among the varieties. Stem height and grain yield do not always provide a reliable measure of straw yield.
Table 33. Straw production of selected wheat varieties in 1997
Varieties |
Calhoun, GA
|
Griffin, GA
|
||
Straw Yield (ton/acre)
|
Height (in.)
|
Straw Yield (ton/acre)
|
Height (in.)
|
|
Jackson |
2.11
|
40
|
2.17
|
41
|
NK Coker 9803 |
1.79
|
40
|
2.30
|
36
|
Pioneer Brand 2643 |
2.06
|
35
|
1.86
|
35
|
Pioneer Brand 2580 |
1.78
|
38
|
2.30
|
38
|
AgriPro Hickory |
1.71
|
40
|
2.10
|
41
|
NK Coker 9663 |
1.62
|
41
|
2.20
|
39
|
AgriPro Mason |
1.44
|
40
|
1.96
|
39
|
NK Coker 9835 |
1.43
|
34
|
1.83
|
34
|
Clark |
1.40
|
37
|
2.04
|
39
|
GA-Dozier |
1.40
|
37
|
2.37
|
37
|
GA-Gore |
1.32
|
38
|
1.91
|
40
|
Pioneer Brand 2684 |
1.30
|
40
|
2.00
|
39
|
Fleming |
0.70 |
31
|
1.93
|
39
|
Table 34. Straw production of selected barley varieties, Calhoun, GA, 1997
Varieties |
Straw Yield (ton/acre)
|
Grain Yield (bu/acre)
|
Height (in.)
|
Nomini |
1.51
|
70.7
|
39
|
Pamunkey |
1.50
|
73.9
|
36
|
Clemson 100 |
1.34
|
41.6
|
38
|
GA-Luttrell |
1.20
|
78.9
|
35
|
GA-Acton |
1.60
|
58.7
|
36
|
Venus |
1.08
|
70.3
|
36
|
Wysor |
0.75
|
37.4
|
36
|
Before removing the straw from the fields, consideration should be given to the benefits of leaving the residue. Removing residue removes nutrients from the field and also reduces potential water conservation for the summer crop by leaving the soil exposed. Each ton of straw contains approximately 12 to 14 lb of nitrogen, 3 to 4 lb of phosphorous, 5 lb of sulfur, 25 lb of potassium, 8 lb of calcium, 5 lb of magnesium, and trace amounts of copper, manganese, and zinc (Source: W.C. White and D.N. Collins, eds., 1976, The Fertilizer Handbook, Fertilizer Institute, Washington, DC). Determining the value of nutrient removal when removing straw from the field should always be considered when determining the economics of straw utilization.
Production systems that promote high grain yield promote good straw production. It is important to check labels of pesticides that are used in grain production so as to be in compliance when choosing to use the residue.
BARLEY
Barley (Hordeum vulgare) is best adapted to the upper Coastal Plain and northern sections of the southeastern states. High temperature and humidity in the lower Coastal Plain favor diseases that greatly reduce grain yields. Barley grows best in well-drained loam and clay loam soils. Light sandy soils are poor for barley, because growth is often erratic and the crop is more likely to ripen prematurely under drought conditions. Barley is unsuited for acid soils that increase aluminum toxicity, resulting in stunted root and top growth.
Barley can produce more than 70 bushels/acre in adapted areas of the Southeast without irrigation. Early varieties such as Venus mature two weeks before the earliest wheat in the Piedmont region, which facilitates the timely planting of soybeans and grain sorghum in doublecropping systems. Barley is grown primarily as a grain crop but may also be used for grazing in the Piedmont and Limestone Valley areas.
Barley is not recommended for winter grazing in the Lower Coastal Plain due to insect pests and diseases. Barley will begin to provide grazing six weeks after planting. Winter barley becomes dormant after four days of sub-freezing temperatures, thus it will not provide as much winter grazing as rye or wheat. If barley is grown for grazing and grain, cattle should be removed before the plants are in the jointing stage of growth so the growing point is not grazed off.
Fertilization
All fertilizer and lime applications should be based on soil test recommendations (soil pH should be between 6.0 and 6.2). Barley following soybeans and corn should receive 20 lb/acre of nitrogen at planting; following grain sorghum, a minimum of 40 lb/acre of nitrogen for grain production. Sixty lb/acre of nitrogen should be top-dressed when the first node appears above the soil surface. If barley is used for grazing, half the nitrogen should be applied at planting and the rest in late winter or as grazing is needed (Table 35).
Table 35. Fertilizer recommendations for barley for the entire growing season
Crop use
|
N
|
P205
|
K20
|
Grain
|
80
|
40
|
40
|
Grazing
|
120
|
50
|
50
|
Tillage and Cropping Systems
Seedbed preparation by deep tillage is necessary to reach maximum yield potential of the crop. Plowing buries crop residues and weeds and aids in disease control. Barley is not as winter hardy as rye or wheat, so it needs to be planted about two weeks earlier than these small grains (Table 36). Continuous planting of barley as a single crop or in a doublecropping system such as soybean-barley is not desirable because of buildup of barley diseases.
Table 36. Optimum planting dates for barley
Region |
Grain
|
Grazing
|
Limestone Valley |
Oct. 1
|
Sept. 15
|
Piedmont |
Oct. 15
|
Oct. 1
|
Upper Coastal Plain |
Nov. 15
|
Oct. 1
|
Planting
Barley should not be planted as deeply as other small grains because the coleoptile, the seedling sheath that penetrates the soil surface, does not elongate as much. Barley should be planted 1 to 1½ in. deep when soil moisture and temperature are favorable for rapid germination and emergence. Barley germinates best when soil temperatures drop below 60°F for a 10-hour period. Grain drills offer good control of planting depth and rate. Broadcast seeders may cause uneven stands due to poor seed distribution. Incorporation of seed with a disk harrow can result in varying seeding depths.
The standard test weight for barley is 48 lb/bu. Plant 2 to 2.5 bushels/acre of seed (96 to 120 lb or 25 to 32 seeds/sq ft) for grain and 3 bushels/acre (144 lb or 38 seeds/sq ft ) for grazing.
Storage
Barley straw deteriorates rapidly after ripening, therefore barley should be combined as soon as the kernel moisture reaches 14 percent. Lodging prior to ripening is a problem especially in the taller varieties. Combine speed will have to be reduced to 2 miles per hour and the cylinder speed increased over that used for wheat or rye (see "Harvesting, Drying and Storage" chapter). Harvest-ing should be done on bright, sunny days when the relative humidity is below 50 percent. Harvested grain should be dried to 12 percent moisture before storing.
Utilization
Barley is an excellent feed grain for cattle, having a feeding value 90 percent that of corn when steamrolled (flaked), crimped or coarsely ground. Cattle do well when fed barley because their digestive systems can handle the hulls of barley. Hulls (13 percent to 14 percent of kernel) detract from the nutritive value of barley when fed to swine and poultry. A ration of 50 percent wheat and 50 percent barley (ground coarsely) is very good for fattening hogs. It produces a firmer, leaner meat than corn because of the lower oil content of the kernel. A mixture of barley and corn can be used also, but protein supplement will be needed as corn has less protein than wheat.
Barley Insects and Diseases
Barley planted early for grazing may suffer damage from bird cherry-oat aphids and English grain aphids. These and other aphids are vectors for barley yellow dwarf virus and may have to be controlled by chemicals if ladybug populations are low. Hessian fly is seldom a problem on barley except when planted very early. Varieties differ in susceptibility to Hessian fly. For information about insect control, see "Insect Management and Control" chapter.
Several important diseases may reduce barley yield 20 percent or more. The most effective control methods are summarized in Table 37.
Table 37. Effectiveness of control methods for barley diseases
Disease |
Seed Quality & Treatment
|
Resistant Cultivars
|
Crop Rotation
|
Foliar-applied Fungicides
|
Cultural Practices
|
Spot blotch |
2
|
1
|
1
|
|
b, c
|
Scald |
2
|
1
|
1
|
1
|
|
Barley stripe |
1
|
2
|
|
|
|
Loose smut |
1
|
2
|
|
|
|
Barley yellow dwarf |
|
2
|
|
|
a, c
|
Leaf and glume blotch |
2
|
2
|
1
|
1
|
b, c
|
Leaf rust |
|
1
|
|
|
|
Stem rust |
|
1
|
|
|
|
Powdery mildew |
|
1
|
|
|
|
Take-all |
|
3
|
1
|
|
a, b, c
|
1 very effective, 2 moderately effective, 3 slightly effective
a delaying time of planting, b deep tillage, c weed control
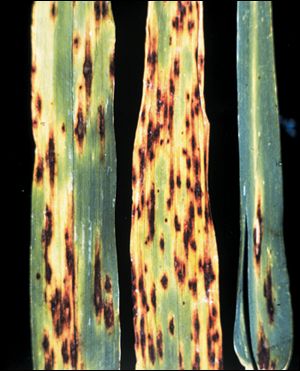
Spot blotch (Figure 28), caused by the fungus Bipolaris sorokiniana (syn. Helminthosporium sativum), is the most important leaf spot disease of barley in the Southeast. It causes a circular to elliptical dark brown to black spot surrounded by a narrow yellow margin. Spot blotch affects the leaves and heads of barley. When heads are infected, the glumes (chaff) attached to the seed and the seed itself are darkened. The disease on seed is called "black point" because the end of the seed is discolored and shriveled. The spot blotch fungus survives in the seed and can cause seedling blight when the seed germinates.
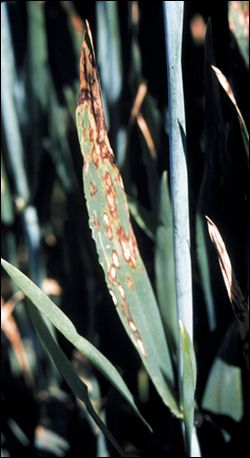
Scald (Figure 29), caused by the fungus Rhyncosporium secalis, appears before jointing on susceptible varieties. The disease is favored by wet weather and temperatures from 60° to 70°F. Spots begin as water-soaked areas, which collapse within one to two days to form elliptical to diamond-shaped spots ½ in. or longer that have a bleached center and dark brown margin. These dried lesions give the leaf the appearance of having been scalded, hence the common name of the disease. When the disease is severe, heads also may be affected which results in seed infection. Crop rotation, resistant varieties, and planting clean seed are effective control measures.
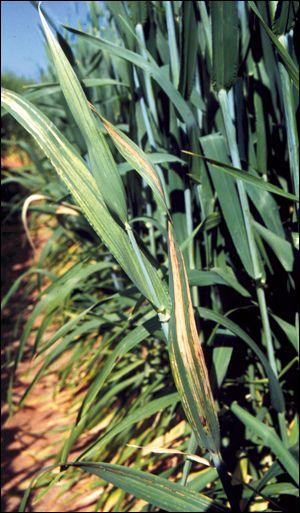
Barley stripe (Figure 30) is caused by the fungus Pyrenophora graminea, which is also known as Drechslera (Helminthosporium) graminea. Flowers are infected in the spring and the fungus invades the developing seed without causing any seed damage. When the seed germinates the fungus grows with the growing point of the young plant. Before the jointing stage, the fungus grows into the young leaves causing faint narrow yellow streaks that extend the length of the leaf. The streaks quickly turn brown and become covered with a dense mat of dark fungus spores. The tissue between the leaf veins disintegrates and the leaf splits lengthwise along several of the stripes. The leaf rapidly dies and becomes ragged and curled. Tillers may fail to produce heads or only heads that are sterile. Only infected seed transmits barley stripe. The disease does not affect other small grains. It is controlled easily by planting certified seed and/or seed treatment with carboxin (Vitavax), triadimenol (Baytan), difenoconazole (Dividend) or tebuconazole (Raxil) (see "Diseases of Wheat" chapter).
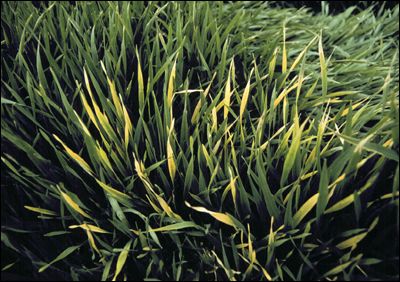
Barley yellow dwarf virus causes a serious disease of barley (Figure 31). Infection occurring in the fall results in intense yellowing beginning on the upper part of the leaves and extending toward the base; plants are often severely stunted.
In the spring, plants are yellow, but stunting is not usually seen. Disease development, control and transmission of the virus by aphids is the same as described for wheat (see "Diseases of Wheat" chapter).
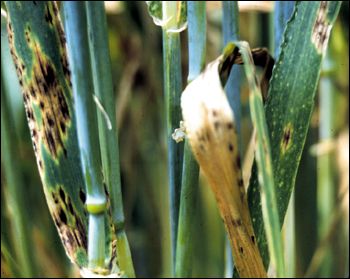
Leaf and glume blotch is caused by a strain of Stagonospora nodorum pathogenic to barley but not pathogenic to wheat. This strain occurs on barley throughout the Southeast, but it is much less serious than on wheat (Figure 32). Spots are elliptical with more yellow around the edge than is usually seen on wheat. When the spot is on the midvein of the leaf, the yellowing often extends beyond the lesion to the tip of the leaf. The center of the spot becomes tan as it gets older, but darker brown streaks remain throughout the spot. Numerous minute dark specks appear in the center of the lesions. These are the fungus' fruiting bodies (pycnidia), which contain the spores. Glume blotch can also develop on heads. Disease development and dissemination of the fungus are identical to that on wheat. The disease is controlled by resistant varieties and crop rotation. Other diseases: Loose smut is caused by Ustilago nuda and is similar to loose smut of wheat (see "Diseases of Wheat" chapter). Leaf rust, stem rust and powdery mildew are seen during wet springs following mild winters, which favor overwintering of the diseases. Losses are rarely significant. Barley is less susceptible than wheat to take-all, and the disease is controlled by rotation. All barley varieties are susceptible to take-all.
OATS
Oats are grown in the South as a grain crop, as a forage crop (greenchop, ensilage, haying and grazing), alone or in mixtures with rye and clovers, as a catchcrop for nitrogen, as a crop to control erosion (cover crop) and as a crop to add organic matter to the soil (green manure).
The palatability and forage quality of oats makes it the best of the small grains for grazing. As a result, the performance of animals pastured on oats (or, more commonly, oats in combination with other winter annual grasses or legumes) is outstanding. The main factor that limits the use of oats as a winter annual grazing crop in Alabama and Georgia is that, in general, oats lack good winter hardiness. But, some varieties are much more winter hardy than others, and cold weather risk is less in the southern, as opposed to the northern, portions of the region.
Adaptability
Although oats may be grown throughout Alabama, Florida and Georgia, they are best adapted to the central and southern regions. Oats are less winter hardy than wheat, barley or rye and will generally suffer yield losses when the temperature falls below 20°F.
Variety Selection
The selection of a variety is an important decision that has consequences for the rest of the growing season. Most farmers consider yield to be the most important consideration in selecting varieties. Other characteristics, though, such as disease resistance, winter hardiness, maturity and straw strength are also important considerations when selecting varieties.
Each year University agronomists conduct oat variety trials at numerous locations throughout the South to determine which varieties are best-adapted, which have the highest grain or forage yield and which have the most desirable characteristics for various purposes. Lists of recommended varieties are compiled annually based on these evaluations.
Additional information on yield, disease resistance and other characteristics of oat varieties can be found in the annual variety performance reports from your state. Copies of the current report and variety recommendations can be obtained at your county Extension office or from the Web.
In addition to selecting a good variety, use good quality, certified seed. If non-certified seed is used, a seed test should be conducted to make certain the seed is free of weeds and has a good germination level.
Seed Treatment
Fungicide seed treatments are an inexpensive and effective method of protecting seed and seedlings from seed and soilborne diseases. Certified seed purchased through a seed distributor is often treated with a fungicide. Seed from local sources often is not treated at all.
A fungicide seed treatment is desirable for use on all seed grown for grain. These fungicides may be formulated for commercial application, as drill-box treatments, or both. Machine-applied products are cheaper and provide better protection than comparable drill-box treatments; however, the availability of commercially treated seed is limited in some areas.
Drill-box treatments can provide good protection from most seed and soilborne diseases. The real key to performance of drill-box treatments is thorough coverage of the seed with the full label rate of the fungicide. To obtain good distribution of the fungicide over seed, fill the hopper half full of seed, add half of the required amount of fungicide to the hopper, and thoroughly mix. Then, add the remaining seed and fungicide to the drill-box and mix until all seeds are covered.
Loose smut can be controlled with the systemic fungicides carboxin or tebuconazole. These products are available in drill-box and machine-applied formulations. Machine-application is considered superior to drill-box treatments.
Planting Dates
The optimum dates for planting oats will depend on the location in the state and the intended use. In general, planting should be completed earlier in the autumn in the northern than in the southern region. Oat plants that are at a more advanced growth stage (tillering) are less susceptible to winter kill than plants at the seedling growth stage. Planting at the recommended planting date maximizes the chances of producing a good grain or forage crop and minimizes the chances of total crop loss through winter kill.
Oats planted for use as forage should be planted earlier than those to be harvested as grain. Small grains planted too late often suffer winter damage and do not yield well. Oats that are planted too early may suffer excessive damage from insects and diseases and may accumulate extra autumn growth that often leads to lodging. If stem elongation and head formation begin in autumn, severe winter kill will usually result. Table 38 lists recommended planting dates.
Table 38. Planting dates of oats for grain and forage production
Region | Grain | Grazing (Prepared seedbed) | Grazing (Planted in sod) |
Alabama | |||
Northern | Sept. 15-Oct. 15 | Aug. 25-Sept. 20 | Oct. 1-Oct. 15 |
Central | Sept. 15-Oct. 15 | Sept. 1-Sept. 15 | Oct. 15-Oct. 30 |
Southern | Oct. 15-Nov. 30 | Sept. 15-Sept. 30 | Nov. 1-Nov. 15 |
Georgia | |||
Mountains | Sept. 25-Oct. 15 | Sept. 1-Sept. 30 | – |
Piedmont | Oct. 7-Oct. 30 | Sept. 15-Oct. 15 | – |
Coastal Plain | Nov. 7-Dec. 1 | Sept. 15-Oct. 15 | – |
Florida | |||
Northern | Nov. 15-Dec. 15 | Sept. 15-Nov. 15 | – |
Seedbed Preparation
Preparation of a smooth, firm, vegetation-free seedbed aids in precise planting and obtaining a good stand. Disking is probably the most common method of seedbed preparation for oats. However, recent studies suggest that some sort of deep tillage before planting may be beneficial, particularly on coastal plain soils. Chiselplowing and turn-plowing usually result in 5 to 15 bushels/acre more grain than disking or no-till and 600 to 1,000 lb/acre higher forage yields. A paraplow or subsoiler with a 30- to 36-in. shank opening is as effective as chiseling or turning.
Planting no-till also can be profitable. The key is depth control. For a good stand, the plant debris from the previous crop needs to be spread evenly, and the use of a no-till planter is essential.
Planting Rate and Depth
Planting Rate
The quantity of seed needed per acre varies in different situations. First, seeding rates depend to a great extent on the intended use or uses of the crop. More seed should be used in fields that are to be grazed than in areas where oats are being grown for grain only.
Secondly, the method of planting also influences the quantity of seed needed. The best method for planting oats is with a grain drill or other drill-type planter that allows precise planting. If the seed are to be broadcast and disked in, increase the seeding rate by 20 percent.
The seeding rate should also be increased with any of the following conditions: late planting, no-till planting, planting into a dry seedbed, or aerial planting. The recommended planting rate for oats planted for grain is 60 to 90 lb/acre. The recommended planting rate for oats planted for forage is 90 to 120 lb/acre. If seeded with ryegrass or clovers, the seeding rate for oats is 60 to 90 lb/acre.
Planting Depth
Oat seed should be placed in firm contact with enough soil to prevent rapid drying. Seed should be placed at depths of ¾ to 1½ in. Deeper coverage may result in loss of vigor and failure of the seed to produce emerged seedlings.
For uniform depth of coverage, the seedbed should be smooth and the grain drill should be adjusted so that all openers are planting at the same depth. If some openers are planting too deep or shallow, poor seed emergence and poor stands may result in those rows.
Liming and Fertilizing
There is no substitute for soil testing in determining how much lime and fertilizer to apply for oat production. When taking soil samples, avoid atypical areas such as depressions or eroded sites. A good fertilizer recommendation depends on a good soil test.
Liming
Soil acidity (low pH) often reduces the yield of oats in the South. This can be easily corrected by liming. The recommended range for oats is 5.8 to 6.5. Any needed lime should be incorporated into the topsoil before planting time.
Nitrogen
For grain production apply 20 lb of nitrogen (N)/acre at planting or soon after seedling emergence. Apply an additional 60 lb N/acre in February for oats behind a summer legume and 75 lb N/acre after a non-legume crop. When oats are planted behind soybeans or peanuts, the autumn nitrogen application can be omitted. Delaying the February nitrogen application until March may severely reduce yields, especially when oats follow non-legume crops. Oats to be grazed should receive 100 lb N/acre, followed by an additional 60 lb N in late winter. If planted with a winter annual legume, or if sod-seeded in late autumn, 60 lb N/acre should be applied near planting. With grass and legume mixtures, 60 lb N may be applied in late winter, depending on whether additional forage is needed. Comparisons of different nitrogen sources have generally shown little or no difference in effectiveness, provided the application requirements of the source used are met. However, gaseous losses from urea or liquids containing urea can be important under conditions of high temperatures, dry soils, high soil pH (7.0 or higher), and where large quantities of plant material cover the soil surface. Under these conditions, ammonium nitrate would be the preferred nitrogen source. To minimize losses, dribble urea nitrogen instead of applying broadcast.
Phosphorus and Potassium
Phosphorus (P) and potassium (K) are important in oat production. Needs vary from field to field, and fertilization with these elements should be based on a soil test.
Magnesium and Sulfur
Available magnesium (Mg) is routinely determined on soil samples at most soil testing laboratories. Where Mg is low and liming is needed, dolomitic lime is recommended. However, on fields that are low in P and high in K and N, problems with grazing cows and sheep are likely, because these circumstances favor Mg-deficiencies in animals. Calcium (Ca) is also supplied by lime and is generally not deficient in areas where there is a suitable soil. Sulfur (S) may be deficient on sandy soils where little or no S has been applied recently. The application of 10 lb S/acre in the form of ammonium sulfate, ammonium thiosulfate, or other sulfate is recommended each year to prevent S deficiencies. Up to 20 lb S/acre may be needed on deep sands to prevent sulfur deficiencies. The best time to apply sulfate sulfur is at topdressing in February.
Micronutrients
Micronutrients are generally available in most soils in adequate amounts for oats production. Therefore, routine applications of micronutrients to oats are not recommended.
Weed Control
Weed control for oats is similar to wheat. However, DO NOT use the herbicide Hoelon for oats, because Hoelon will kill oats. Consequently, annual ryegrass can not be effectively controlled with herbicides in oats.
Disease Management
The following are the most common diseases for oats in the South:
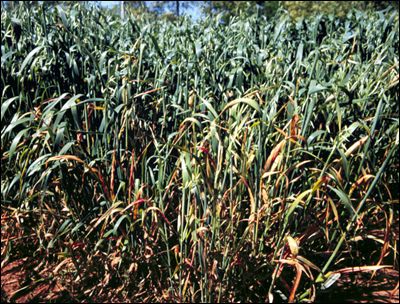
Barley yellow dwarf is caused by the same virus that causes yellow dwarf on barley and wheat. However, oats are more susceptible and severely damaged by this virus than wheat. The disease is often called "red leaf disease" because leaves of infected plants become uniformly red from the tips backward. The virus is spread by several kinds of aphids. When infection occurs early in the season, plants are stunted and produce no or few heads. Postponing seeding until the latter part of the recommended planting period may decrease the incidence and severity of this disease (Figure 33).
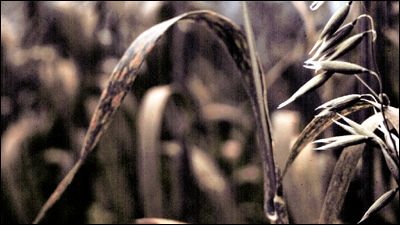
Crown rust, caused by the fungus Puccinia coronata, is one of the most destructive diseases of oats. It is recognized by flaky red postules on the leaves, and it is similar to leaf rust in wheat. Because crown rust can develop rapidly when environmental conditions are favorable, it can cause serious yield losses. Varietal resistance is the primary means of control, but fungicides may be effective when rust is severe (Figure 34).
Helminthosporium leaf blotch, caused by the fungus Drechslera avenae, attacks both leaves and kernels. Oval, reddish-brown spots (sometimes with a white-gray center) are common symptoms of this disease on seedling leaves. Diseased kernels turn brown at the basal stem. By attacking the leaves, photosynthesis will be reduced, resulting in light and shriveled kernels. This happens also by direct attack of the fungus on the kernels. Crop rotation, variety choice, and fungicide treatment on the seed will reduce this disease.
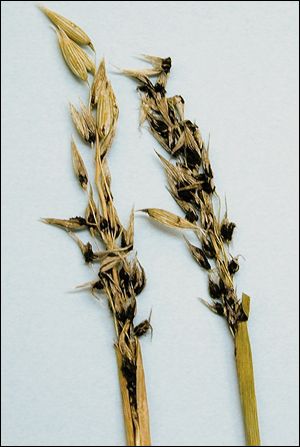
Loose smut, caused by the fungus Ustilago avenae, causes blackened heads similar to that of loose smut of wheat and barley (Figure 35). However, the loose smut fungus does not invade the embryo, like U. tritici on wheat and barley. Spores lodge on the seed and germinate to infect the oat seedling. The fungus then grows systemically in the plant into the floral tissues. Certified seed must have less than 0.5 percent smut as determined by field inspection.
For more information about these and other diseases, see Alabama Cooperative Extension System circular ANR-631, Oat Diseases in Alabama, which has color photographs that are useful in identifying disease problems.
Insect Management
Insect pests and their control are basically similar to that as for wheat, except oats are immune to the Hessian fly. Conversely, cereal leaf beetle usually prefers oats to any other host, but if not given a choice, will readily feed on all small grain crops.
Grain Harvesting and Drying
Oats should be harvested as soon as the grain moisture content drops to 13 percent. Rain or dew will cause discoloration of the grain and will lessen the marketability of the crop. Combining at high moisture (15 percent) and artificial drying may be warranted if kernel brightness is important. If drying is necessary, temperatures no higher than 110°F should be used for oats intended for seed. Oats to be fed can be dried at temperatures up to 200°F. The minimum air flow rates and maximum first-layer depths for in-storage drying are in Table 39.
Table 39. The maximum air flow rates and maximum depth of first layer of in-storage drying oats at different moisture contents
Moisture content(%)
|
Air flow required (cfm/bu)
|
Max. depth of first layera (ft)
|
Approx. static pressureb(in.)
|
20
|
2.5
|
6
|
1.9
|
18
|
2.2
|
7
|
2.3
|
16
|
1.5
|
8
|
1.7
|
14
|
1.0
|
10
|
1.8
|
a Depths must be reduced if grain contains fines.
b Pressures given include 0.25 in. for duct losses.
RYE
Rye was originally cultivated in southwestern Asia during the first millennium B.C. It is a member of the grass family and only one species of rye, Secale cereale L., is extensively cultivated. Worldwide, rye is used as forage for livestock pasture, grain for livestock feed and flour for human consumption. Rye also is used as silage, green manure and a cover crop. It is grown extensively in the Southeast for winter forage and is adapted to most areas. Georgia has led the nation in planted rye acreage for many years, averaging more than 300,000 acres in recent years. Rye is seeded, either as pure stands or in mixtures with ryegrass or other winter annuals. Although the majority of it in the South is used for grazing, rye also is used as a grain for feed and for seed production and as a cover crop. It produces high-quality forage during the cool-season months when forage from perennial warm-season grasses is limited. Rye produces more forage early in the season, whereas wheat and oats produce most of their forage later in the season. It is the most dependable cereal species for winter grazing in this region due to its greater cold tolerance, higher growth rate at low temperatures, and more uniform seasonal forage distribution in comparison to wheat, triticale and oats.
Rye has a number of advantages over other crops. It is considerably more winter hardy than other cereal crops and will give economic yields on marginal soils. It produces an extensive root system and is more tolerant to acidic, light, or sandy soils than other cereal crops. Rye is a good rotational crop and fits well in doublecropping systems.
Cultivar Selection
Factors that should be considered when choosing a rye cultivar for winter forage production include forage yield, dependability of forage production, seasonal distribution of forage production, pest resistance, and forage quality. Characteristics necessary for high and stable winter forage production in the Southeast include high forage yield potential, cold tolerance, Hessian fly resistance, and the capacity for rapid regrowth and recovery from stress conditions. Current data for cultivar characteristics and grain and forage performances are available in annual research reports published by experiment stations and are available through the Cooperative Extension Service.
In selecting cultivars, give attention to varietal characteristics and performance in the production region where the crop is to be grown. Early maturing ryes will produce more early season forage but have shorter production seasons. Medium and late maturing ryes will provide a longer grazing period. Rye cultivars with different growth habits can be blended to provide better forage distribution and higher forage yields.
Cropping Systems
Rye fits well in doublecropping systems as a forage crop and a feed grain, and may improve growers cash flow because it brings money at a different time of the year than many other crops. Rye is often planted after corn, peanuts, soybeans and tobacco. It is very efficient in utilizing residual fertilizers applied to previous crops.
Doublecropping with soybeans and peanuts after harvesting rye as forage is feasible in the Southeast. If rye follows a legume, such as peanuts or soybeans, the total amount of nitrogen applied to rye can be decreased by 20 lb/acre. Rye also can have a beneficial effect as a cover crop for vegetables, cotton, tobacco, and peanuts. To avoid buildup of harmful insects and seedling diseases, rye should be grown only once every three years on the same land if possible.
Planting Dates
Optimal planting date varies with location. Planting before recommended planting dates does not insure better forage yields and sometimes results in increased problems. Late planting of rye is desirable if early grazing is not needed or if the crop is intended only for grain production. Suggested planting dates in the Southeast for forage production and grain yield are shown in Table 40.
Table 40. Planting dates for rye
Location
|
Forage
|
Grain
|
Mountains
|
Sept. 1
|
Oct. 15
|
Piedmont
|
Oct. 1
|
Nov. 1-Nov. 15
|
Coastal Plain
|
Oct. 15
|
Nov. 7-Dec. 1
|
Land Selection and Seedbed Preparation
One consideration for winter grazing is the land available for winter pasture. Good surface drainage is absolutely necessary to keep the winter crop growing and to give the greatest number of grazing days. Rye gives highest yields on fertile well-drained soils and does not yield as well on soils that are low in fertility and water holding capacity. Well-drained, slightly rolling fields produce the most forage and usually the greatest number of grazing days. Rye is adapted to soil types throughout the Southeast, but wet, improperly limed, and poorly fertilized areas should be avoided. Rye is the most drought-resistant of the cereals because of its extensive root system. The extreme winter hardiness of rye and its ability to grow in low-fertility soils makes it an alternative crop for some areas.
Deep tillage, such as moldboard, chisel or parabolic subsoiler, is recommended for seedbed preparation. This is especially true for Coastal Plain soils, which tend to form hard pans. It also can be beneficial to bury trash and litter from the previous crop. This may reduce problems with soilborne diseases and insects.
Seeding Rates
Cleaned, treated, high quality and preferably certified rye seed should be planted at rates of 1 to 1.5 bushels/acre (56 to 80 lb/acre) for grain production and 2 to 2.5 bushels/acre (112 to 140 lb/acre) for grazing purposes. One pound of rye seed typically contains 24,000 seed, but seed weight and volume can vary depending on variety and the environment in which the seed were grown. Thus, a more accurate means is to plant 20 to 24 seeds per linear foot of row with 7 in. between rows for grain and 40 to 50 seeds for grazing. If planting date is delayed or planting conditions are poor, seeding rates should be increased by 15 to 20 percent. Broadcasting seed and disking are less desirable but can be used if a grain drill is not available. Seeding rates should be increased by 20 percent to allow for variable seed placement. If aerial seeding into standing soybeans is practiced, then about 125 lb/acre should be planted.
Planting Methods
Rye should be planted with a grain drill at a depth of 1 to 1.5 in. Care should be taken not to plant or cover the seed too deeply. Planting with a grain drill results in more uniform depth and rate of planting. Broadcasting seed with spreader equipment and disking the field are less desirable but can be used if you have limited drill-planting equipment.
Aerial seeding has been used to plant rye and ryegrass for winter grazing in the Southeast. This method will speed up the planting process on large acreages, by reducing the number of trips over the land. Several factors should be controlled to get satisfactory results. This method can be used following soybeans by seeding just as the soybean leaves turn yellow. The soybean leaves then drop, covering the rye seed. Adequate moisture is necessary for establishment of rye planted in this manner.
Sod-seeding
Rye can be sod-seeded into permanent bermudagrass or bahiagrass pastures to provide additional high quality grazing in late winter and spring. Overseeded crops are generally more successful and require less land preparation when planted into bermudagrass sod rather than into bahiagrass sod.
Successful overseeding of rye into grass sods is dependent on 1) removal of excess growth of the summer crop before seeding of the rye, 2) placement of rye into good contact with the soil, 3) adequate soil moisture to allow rapid germination and seedling establishment, and 4) adequate plant nutrients. Rye seed must be placed in contact with the soil, and the soil firmed around the seed. After excess summer growth has been removed through close grazing, mowing or haying, prepare the seedbed using two passes with a tandem disk set for shallow penetration. Extensive disking or disking too deep can cause some stand loss in the grass pasture. Rye seed can be broadcast onto the disked sod and covered lightly with a drag or roller.
If moisture is adequate, grain drills or sod-seeding drills can be used to overseed sod pastures with less seedbed preparation. A seeding rate approximately of 2 to 2.5 bushels/acre is recommended when overseeding grass pastures.
Overseeded rye should be fertilized with 40 to 60 lb/acre of nitrogen immediately after seedling emergence. Nitrogen should not be applied to overseeded rye at planting, because it may stimulate growth of perennial grass and cause a reduced stand of rye. Limited grazing can begin as soon as the rye is well established and has accumulated several inches of growth. Heavy grazing should be delayed until around mid-January when the rye is growing rapidly. Topdress an additional 60 to 80 lb N/acre in early February for good forage production. Excess growth should be removed in late spring to allow normal development of the perennial grass crop.
Rye grown in combination with ryegrass gives earlier fall grazing and increases the total grazing days on a given field. In a rye-ryegrass combination, the rye contributes most during the fall and through midwinter. Seeding ryegrass into rye pastures can also be used to extend the grazing period, because ryegrass matures in late spring. To gain the advantage of the earliness of the rye pasture and the persistence of the ryegrass, it is often advisable that rye pastures be grazed heavily in December and early January, thus allowing ryegrass planted alone to develop good growth before grazing begins in late winter.
Liming and Fertilization
Rye can be grown on most soils that are well drained, properly limed and fertilized. A soil test is needed to determine the phosphate and potash requirements for your soil. Collect a representative sample and give full information to the testing laboratory on the production level you want to achieve. If no soil test results are available, apply 500 lb/acre of 5-10-15 or a similar grade fertilizer to meet the requirements of the crop.
Lime applications should be based on soil test recommendation. Dolomitic limestone should be used to adjust soil pH to the desired pH range of 5.6 to 6.0, because it furnishes calcium and magnesium and may eliminate potential problems with grass tetany in cattle.
Rye pastures should be fertilized with 120 to 180 lb N/acre. Nitrogen can be applied either as one-half at planting and one-half in late January, or as one-third in each of three applications at planting, in December, and in late January or early February. For grain production, a total of 60 to 80 lb N/acre is adequate, applying 20 to 40 lb N/acre at planting with the remainder topdressed in mid-February. Rye intended for both grazing and grain production should be fertilized with a total of 160 lb N/acre in split applications. The final nitrogen application should be delayed until after grazing is terminated in mid-February.
Grazing Management
Begin grazing when rye plants reach about 6 in. high. Grazing too early reduces forage production and causes serious shortages later in the winter. Extended periods of extremely cold, wet weather or dry periods can cause a shortage of forage. Grazing the crop shorter than 2 or 3 in. in cold weather damages the stand and slows regrowth. This can reduce total animal gains and returns. Green leaves should remain below the grazing height.
Stocking Rate
Rye can furnish between 120 and 140 days of winter grazing. Rye forage is high in quality, containing up to 24 percent protein and 70 percent total digestible nutrients early in the season. Rye pastures will carry one cow-calf unit or 1 to 1.5 steers per acre under normal conditions. Steer gains of 1.7 to 2.0 lb/day have been achieved. When rye pastures are first stocked, there will appear to be a surplus of forage, but a reserve is necessary during colder periods when plant growth is slow. Do not allow crops to grow too tall before grazing, since forage will be wasted and rye may be damaged by cold weather.
Grain Production Following Grazing
Some producers graze rye forage during the fall and winter and then harvest grain in the spring. In Georgia, rye grain yields averaged nearly 1.4 million bu/year during 1993-1995 with the average yield being 23.7 bushels/acre. Standard test weight of rye is 56 lb/bu. If rye is utilized both for forage and for grain production, grazing of pastures should be terminated by early February in the Lower Coastal Plain and by March 1 in the Upper Coastal Plain and Piedmont to allow time for normal development of the seed crop. Grazing after stem elongation begins should be limited because grain yield will be reduced.
Rye heads seldom fill completely. Because rye is a cross-pollinated crop, outcrosses account for most of the seed sets. Rye florets remain open for several days and continuation of unfavorable conditions for cross-pollination result in empty florets. In addition, halo blight kills many individual florets or entire heads in most years.
Rye should be combined as soon as the seed is fully mature when the moisture content is below 14 percent in the field. Safe rye moisture levels are 13 percent for short-term storage. Grain moisture content should be 12 percent or less for safe, long-term storage.
Although used as a livestock feed, rye is a relatively low quality feed grain. It tends to form a sticky mass in the animals' mouths. It is generally used in mixture with other grains.
Insect Pest Management
Cereal leaf beetle, Hessian fly and chinch bug all attack rye. However, Hessian fly is less likely to attack rye than wheat and rarely causes extensive damage to rye. Manage these pests as in wheat, but check insecticide labels carefully. Sometimes an insecticide is labeled for wheat but not rye.
Because rye is planted early for grazing, perhaps the greatest threat to rye is the fall armyworm (see Figure 20). Check young stands shortly after planting, particularly after a dry summer. Young seedlings are vulnerable to damage by this pest. Treat with an insecticide if there are more than two larvae per linear foot in seedling rye.
Rye is often interplanted in pastures and hayfields as a winter forage. In this situation, rye is vulnerable to attack by green June beetles. Green June beetles tunnel through the soil in search of their favorite food, decaying organic matter. In the course of tunneling, rye seedlings are easily tossed out of the ground. Green June beetle infestations are most likely to occur in fields in which broiler litter or manure has been used as an organic fertilizer. Scout pastures in the fall, before rye is planted, for thinned areas of pastures or trails of pulverized soil. Dig in suspect areas to see if green June beetle grubs are present. Green June beetle grubs are easily recognized because they crawl on their backs. If more than one green beetle grub is found per square foot, treat with the appropriate insecticide before planting rye.
Weed Management
In cases where winter broadleaf weeds become a problem, they can be controlled by application of amine 2,4-D or a similar herbicide once the grazing crop is past the seedling stage. Herbicides should not be applied to rye in the seedling or boot to heading stages. Herbicides should be applied to rye in accordance with label recommendations. Failure to do so will result in increased risk of crop injury. Rye treated with certain herbicides should not be grazed or harvested as forage. Contact your county agent for current herbicide recommendations. Information can also be obtained from the Georgia Pest Management Handbook or Alabama Extension IPM-0458, Small Grain Insect, Disease, and Weed Control Recommendations.
Disease Management
- Seedling blights can cause significant reductions in the stand of rye, especially when planted for grazing in late summer when soil temperature is still high. Several species of Pythium fungi that reside in soil cause both pre- and post-emergence damping-off. Damage is most severe in the lower coastal plain where these high-temperature tolerant species occur. As soil temperature declines below 77°F, seedlings are able to emerge quickly and complete the early growth stage when they are most susceptible. Daily soil temperature data throughout Georgia can be accessed through the Environmental Monitoring Network (check the University of Georgia CAES Web site at www.caes.uga.edu for information). Seed treatment with the fungicide metalaxyl controls Pythium. It is often included with fungicides that control other seedling fungi and smuts. These treatments may be more expensive than other seed treatment fungicides but should be considered for plantings in August through September in Coastal Plain areas. Proper seed treatment reduces the number of disease-causing organisms on the surface of the seed and the loss due to seedling diseases. Apply recommended seed treatments according to label instructions unless purchased seeds have been treated (see Georgia Pest Management Handbook).
- One of the most important and often overlooked diseases of rye in the Southeast is halo blight caused by the bacterium Pseudomonas syringae pv. coronafaciens. Halo blight is also common on oats but usually causes only minor damage in the early spring. Symptoms vary significantly depending on the time of the season. The bacterium produces a toxin that causes a bright yellow halo when temperatures are cool in early spring. The spots are usually circular but may extend to the leaf tip if infection occurs in the midvein of the leaf. The tissue collapses rapidly and dies. Damage to leaf tips in early spring looks very similar to frost damage. As temperatures increase symptoms become small circular spots which have a dull straw color. These "scald" lesions may extend across the entire width of the leaf sheath, killing the entire leaf blade. This symptom is often attributed to maturity rather than to halo blight. Scald lesions may be scattered on the leaf blades late in the season. If infection occurs when the head is in the whorl, the entire rye head may be blighted. When individual florets are blighted, the flowers die and turn white. Adjacent florets may be healthy and produce normal seed. The disease is difficult to control on rye because most cultivars are very susceptible. The bacterium survives on plant surfaces of many plants and thus occurs in all fields. Bactericides are not available for control. Crop rotation is most effective.
- Leaf rust can damage rye. Symptoms are the same as on wheat (see Figure 8). Genetic resistance is the primary means of control.
- Leaf and glume blotch occurs on rye and causes the same symptoms as on wheat (see Figure 7).
- Smuts and powdery mildew rarely affect rye.
TRITICALE
Triticale is a cool-season annual small grain crop developed by crossing rye with wheat in an attempt to combine the high grain yield and quality of wheat with the hardiness and high lysine content of rye. Crossing durum wheat with rye developed the best triticales adapted to the Southeast. This type of triticale does not have the functional properties of common wheat and thus far very little has found its way into our food market channels. It is an excellent feed grain and has been used by livestock producers as an energy source in their rations. Because triticale has a better amino acid profile that corn, wheat, sorghum and other energy sources, it also can replace some of the protein supplementation required in animal feeds.
Current triticale varieties produce yields similar to wheat, are 6 to 8 in. taller than most wheats, and have long nodding heads with long, rough awns. Triticale grain is usually larger than wheat, but tends to be wrinkled or shriveled. The standard bushel weight is 48 lb/bu. Under good growing conditions, test weights can be in the 52 to 54 lb/bu range. The grain is softer than wheat with a more pointed embryo and is more easily damaged if the combine isn't adjusted properly.
Variety Selection and Planting Date
Beagle 82, Florida 201 and Florico are older varieties that have done well in the Southeast. Sunland, developed by the Florida and Georgia experiment stations; Trical Brand 498, developed by Resource Seeds Inc.; and Arcia, developed by the North Carolina Experiment Station, are newer, better performing varieties and should replace the older varieties. All these varieties are spring types, which means they have a low vernalization requirement. They should all be planted in the second half of the recommended planting date period for wheat. If planted too early, they will joint or head too early in the spring and be damaged by late season freezing temperatures. They are not as winter hardy as rye or wheat but will take fairly cold temperatures if they are in the vegetative growth stage. Because of this, triticale is recommended only in the Coastal Plain region.
These varieties are all grain types and do not perform well in livestock grazing systems, because they do not regrow well when defoliated. They have an upright growth habit and do not tiller as well as wheat. They will do quite well as one-cut silage crops and are quite valuable to dairy operations. These varieties all have a low vernalization requirement; if planting is delayed by bad weather or other circumstances, they can be planted from December 15 to January 15 and still produce a useful crop, although yields will be reduced.
Seeding Rate and Planting Depth
Triticale does not tiller as well as wheat and does not compensate as well for poor stands. Plant at least 100 to 120 lb/acre of clean, high quality, weed free triticale seed to ensure an adequate stand. Plant triticale 1 to 1½ in. deep into moist soil. Also, plant triticale with a grain drill into a prepared seedbed if possible. Broadcasting and harrowing will produce a crop, but the yield is often decreased. If triticale is broadcasted and disked in, increase the seeding rate by about 20 percent above what would be planted with a grain drill. A no-till drill also can be used but make sure that you get good seed to soil contact and use slightly higher seeding rates.
Fertility
Triticale seems better able to tolerate unfavorable soil conditions than wheat. In particular, it competes better than wheat on sandy, acid soils. Good yields, however, depend on balanced nutrition and a soil pH in the range of 5.8 to 6.5. This will prevent problems with aluminum and manganese toxicity, and with few exceptions, will ensure adequate availability of boron, copper, iron, manganese and zinc.
The total amount of fertilizer-nitrogen recommended for triticale grain production is the same as for wheat but avoid applying more than 40 lb/acre of nitrogen at planting. Higher nitrogen rates at planting can increase susceptibility to freezing temperatures and thus result in significant winter killing. Late nitrogen applications will increase the triticale protein content. The nitrogen top dressing should be applied a week or two earlier than that for wheat, because triticale normally starts jointing earlier. The nitrogen needs to be applied early enough to encourage tillering and tiller survival. Also, earlier nitrogen top dressing will encourage a larger head and seed size. If the soil is sandy, it may be necessary to add 10 to 15 lb/acre sulfur with the nitrogen top dressing.
Harvesting
Triticale is prone to preharvest sprouting and should be harvested as soon at the grain moisture content drops to 13 percent. Harvesting and drying methods for triticale are similar to those of wheat. However, triticale is more difficult to thresh and more prone to kernel breakage. If possible thresh triticale later in the day when heads are dry and brittle.
Marketing
The markets for triticale are not well developed because of the small acreage. You can not just deliver your triticale to a grain elevator. There is good demand for the grain among swine feeders, and the grain is quite often fed on the farm where it is grown. It is difficult to arrive at a price for the grain, but it is worth about 105 percent to 110 percent the value of corn as a feed grain, because of its higher protein and lysine content than corn. Triticale can substitute for all the corn and part of the soybean meal protein supplement in a swine ration. It is very much prized by swine feeders because it comes in at a time of the year when corn supplies are low. Currently, there is no interest by the milling and baking industries in triticale.
Triticale Pest Management
Triticale is susceptible to most of the same diseases and insect pests which attack wheat.
Two major leaf diseases of triticale are leaf rust and leaf and glume blotch. Symptoms of both are the same as on wheat (see figures 7 and 8).
Bacterial stripe or black chaff, caused by Xanthomonas campestris pv. translucens, also causes some damage to triticale, particularly during wet weather (see Figure 11). The bacterium may be carried on the seed, which is an important means of survival and spread of the disease. Resistant varieties, rotation, and planting clean seed harvested from fields with little disease are effective control measures. Powdery mildew rarely occurs on triticale and is unlikely to be a problem on the crop.
Loose smut and seedling blights are the same as those of wheat. Therefore, seed treatment is recommended. Barley yellow dwarf symptoms have been seen on triticale, but the importance of this disease is not known. Triticale varieties are likely to be more resistant to take-all than wheat, but the fungus strains that infect wheat also attack triticale. Therefore, rotation with oats or a crop other than a small grain is necessary if take-all becomes a problem. Other control measures are the same as for wheat.
Little research has been conducted on triticale insects; consequently, our knowledge of the impact of insects on crop production is limited. The most important insect pest in the Southeast is the Hessian fly, Mayetiola destructor (see Figure 16). The Hessian fly damages triticale primarily by killing seedlings and vegetative tillers during the fall and winter. None of the currently available varieties are highly resistant, but Sunland has suffered less damage than most currently available cultivars. Other insects pests of triticale such as aphids, armyworms, etc. occur sporadically. Insects should be managed in triticale following the guidelines developed for wheat. It has been noticed that stored grain insects are more of a problem on triticale than the other small grains, so it is important to protect the grain if it is going to be stored for more than two or three months.
1Dept. of Entomology
Georgia Station
University of Georgia
Griffin, GA 30223
2Dept. of Plant Pathology
Georgia Station
University of Georgia
Griffin, GA 30223
3USDA-ARS
Auburn University
Auburn, AL 36849
4North Florida Res. & Educ. Center
University of Florida
Quincy, FL 32351
5Dept. of Crop & Soil Sciences
Georgia Station
University of Georgia
Griffin, GA 30223
6Edisto Research & Education Center
Clemson University
Blackville, SC 29817
7Georgia Seed Development Comm.
S. Milledge Ave.
Athens, GA 30605
8Extension Entomology
Auburn University
Auburn, AL 36849
9Extension Plant Pathology
Auburn University
Auburn, AL 36849
10Dept. of Agric. & Applied Economics
University of Georgia
Athens, GA 30602
11Dept. of Biological & Agric. Engineering
University of Georgia
Athens, GA 30601
12Dept. of Entomology
Rural Development Center
University of Georgia
Tifton, GA 31793
13Dept. of Crop & Soil Sciences
Rural Development Center
University of Georgia
Tifton, GA 31793
14Georgia Crop Improvement Assoc.
2435 S. Milledge Ave.
Athens, GA 30605
15University of Florida
Dept. of Plant Pathology
1421 Fifield Hall
Gainesville, FL 32611
16Dept. of Agronomy
Auburn University
Auburn, AL 36849
17Louisiana State University
Cooperative Extension Service
212 Macon Ridge Rd.
Winnsboro, LA 71295
18Dept. of Agronomy and Soils
Clemson University
Clemson, SC 29634
19USDA-ARS- Cereal Rust Laboratory-Southern Unit
Georgia Station
Griffin, GA 30223
20Dept. of Agronomy
Korea University
Seoul, 136-701 Korea
Status and Revision History
Published on Apr 29, 2009
Published with Full Review on Apr 30, 2013
Published with Full Review on Apr 14, 2017